Issue:September 2016
PARTICULATE IDENTIFICATION - Particle Identification for Improvement of Pharmaceutical Production
ABSTRACT
Analyses, including identification, counting, and sizing of unwanted particles in pharmaceutical products is necessary and important and can be very beneficial for product quality and process improvement because it can lead to elimination of specific particle sources. Particles can be found in products, vials, syringes, IV bags, stoppers, production equipment, swabs, scoops, and filters. Collection and preparation methods are very important to the particle analyses. The methods include washing out and filtering as well as physical separation, and must be done in clean environments to prevent contamination. For identification, it is important to consider what needs to be determined with the aspects of the various analysis techniques, including capabilities, technique complementarity, interpretation, speed, ease of analysis, accuracy, and spectral libraries. In this paper, we discuss those aspects with regard to microscopic particle counting, sizing, and identification using Raman, laser-induced breakdown (LIB), and IR spectroscopies. We discuss how those techniques can be used to accurately identify particles, and discuss important aspects to consider when using these techniques.
INTRODUCTION
Good quality of pharmaceuticals requires understanding and control of particulate matter contamination.1,2 Particle characterization and identification in pharmaceutical production can be very beneficial for product and process improvement, and preventing product recalls.3 Particle identification allows possible sources to be determined so that the processes can be improved to prevent unwanted particles and allows production of better quality and safer products, eliminating waste and improving throughput as well as preventing patient adverse effects.
The draft of USP <1790> focuses on detection and removal of product units that show evidence of visible particles. It promotes the need for preventing particulate contamination, which can only come from identification. “No inspection process (manual or automated) can guarantee complete removal of all visible particulate matter or other visible defects; thus, prevention of such defects is an important consideration.”4
Analyses of particles in drugs will improve defect prevention. Analyses of particles from processing equipment will help to improve processing and cleaning. Analyses of particles on process filters can help improve processes and raw material and product specification or purification.5 Analyses of environmental contaminants can help direct and improve personnel training and cleaning and operating procedures. Unwanted particles and materials include hair, fibers, starch, minerals, insect parts, inorganic and organic materials, microbes, stainless steel, cellulose, glass, rubber, polymers, fabrics, and lubricants, among other things. Glass and metals are most critical in terms of product recall.
This paper describes analyses of particles using filtration and manual isolation methods and identification using Raman, laser-induced breakdown (LIB), and IR spectroscopies. We provide information about the important aspects to consider when using these techniques, including capabilities, complementarity, interpretation, ease of analysis, accuracy, and spectral libraries.
MATERIALS & METHODS
The first step of particle analysis is the reliable isolation of particles. Visible particles were removed with cleaned probes. Samples with sub-visible particles or many particles were filtered onto gold filters for direct analysis using Raman, LIBS, and IR.6 Samples were prepared in a Laminar Flow Biohazard Hood, Heraeus Herasafe Kendro HS12 Type A/B3 Class II clean bench.
Raman and LIBS analyses were done with the Single Particle Explorer (SPE) ls metal.ID + raman.ID, manufactured by rap.ID Particle Systems GmbH. For Raman, a 785 nm laser with ≤ 50 mW was used with a spectral range of 300 to 2,000 cm-1 and 5 cm-1 resolution. For LIBS, the pulsed laser was set for 100 μJ, 3 ns. The spectral resolution was 1 nm, and the range was 350 to 800 nm. For Raman spectra, the instrument parameters were ≤ 50 mW 785 nm, spectral range 300 to 2,000 cm-1, and spectral resolution 5 cm-1. Standards libraries and Pearson’s algorithm were used for spectral identification.
FTIR analyses were done with a Thermo Scientific Nicolet iN10 Infrared Microscope using a spectral range 4000 to 675 cm-1 with resolution 4 cm-1. Attenuated total reflectance (ATR) spectra were taken with a Ge ATR attachment. Standards libraries and correlation searching were used.
RESULTS & DISCUSSION
Particles From Vials, Syringes & IV Bags
An example of identification of particles for root cause defect prevention was analysis of a fiber in a vial. The fiber image indicated it was smooth and not twisted, which is typical of polymer fibers, and Raman analysis indicated polyethylene terephthalate (PET). PET can have many sources, and some of the PET materials from the product supplier that were analyzed for comparison included wipes and bonnet and lab coat threads (Figure 1). Even though there were several possible sources of the fiber, identification eliminated several other sources of materials, such as hair or cellulose.
A particle taken from liquid from a syringe was determined using LIBS to be glass with sodium (Figure 2). Though Raman and IR can identify particles as glass, LIBS is particularly effective in determining the source of glass because it can determine the elemental composition. The physical appearance of particles can provide additional information about the source. A fiber may be from fibrous glass insulation, whereas flakes are more typical of syringe and vial glass delamination.
A primary contributor of particulate matter in vial presentations is the elastomer closure due to silicone oil on the surface.1,7 Cellulose is a common contaminant on stoppers. Analysis by Raman and/or IR of many particles on the rubber part of a plunger taken from a filled syringe identified quartz, silicone oil, clay, protein, cellulose, and nylon. In this case, not every particle needs to be identified to confirm the stoppers were not protected from environmental contamination some time during the entire process from manufacturing of the stoppers through syringe filling. It is important to understand if the stoppers are supplied contaminated or become contaminated during the process of manufacturing of the syringes or pharmaceutical product.
IV bags and the infusion sets used to administer the contents can have particles even without any drugs added to them. Table 1 lists the sizes of the numerous particles found in two sequential 10 mL portions taken from an IV bag of 0.9% sodium chloride injection USP 50 mL through an infusion set that had a filter. The two 10-mL portions were filtered onto a gold filter for particle counting and analysis. The filter in the IV bag clearly did not prevent small particles from coming out with the liquid. The number of particles was reduced by about 40% for the second portion removed, indicating the filter may have some effect, or the particles were from the tubing below the filter.
Particles From Process Equipment
Swabs and scoops are often used to determine whether pharmaceutical processing equipment has been adequately cleaned. The particles and materials picked up on these can be analyzed by spectroscopic techniques. The particles can be analyzed directly on the devices, removed and placed on more suitable substrates, or removed into a liquid followed by filtering. Once the particles and materials are analyzed, then the process equipment cleaning protocol can be reviewed and improved to prevent process contamination from those materials. Figure 3 shows the image of a particle removed from a swab and its spectrum identifying it as polytetrafluoroethylene (PTFE) with silicone. The combination may help determine the specific source as tubing, washers, or seals. Then the process can be improved by implementing more frequent checking or changing of fittings so they do not degrade into the process.
A mass of fibers collected from a process on a scoop was analyzed and illustrates the importance of analyzing multiple areas of materials. Raman spectroscopy can analyze small areas of materials, and in this case, polypropylene with white filler as well as cellulose fibers were identified. The polypropylene with filler was consistent with beard cover fabric from that plant.
Filters
There are many types of filters used to collect particles. Figure 4 shows one example of a collodion filter, which is low-nitrated cellulose. LIBS was used to directly identify a particle on the filter as aluminum, which eliminates any steel equipment as a source.
Information Required
The information required to improve the process should direct the analysis. Because every vial and syringe is checked for particles according to USP regulation, it is important to track the number and sizes so that it can be determined if the process is going well. An increase in the number of particles would indicate some process problem. Visible checking only consistently detects particles about 100 μm, and fibers typically have to be much longer to be detected. Knowing the sizes and identification of the particles are essential to understanding the sources, and will lead to understanding how to improve the processing. Thus, techniques that can count, size, and identify the particles should be used. Improvements can include checking and/or cleaning equipment more often, changing parts that wear out before they create particles, perhaps even changing the equipment type, improving cleaning, and better training.
IDENTIFICATION ASPECTS
Methods of Removal
There are methods for analyzing particles in situ. These can work well, but can be complicated by bubbles and contributions from the surrounding materials. Isolating the particles by filtering has the advantage of removing any materials that can interfere with the analysis. Filtering avoids manual isolation, which is difficult, time-consuming, and potentially destructive to the particles. This avoids tedious manual isolation and difficult manipulation of single particles. For filtering particles, it is important to choose a filter with the right pore size and that is tolerant to any solvent used to wash away the supernatant. Gold-coated filters are very good for taking Raman and IR spectra of particles because they reflect the light and enhance the signal. IR reflectance spectra of particles on a gold-coated filter require no extra sample preparation or moving, and spectra of microparticles on gold typically have excellent signal-to-noise. In addition, attenuated total reflectance (ATR) spectra can be taken of the particles if the reflectance spectra are not very good or are significantly different from the library spectra, making spectral identification difficult. LIB spectra can also be taken on gold filters for particles that are about 10 μm.
It is important to consider what analytical technique would be appropriate for identification. LIBS analysis can provide detailed elemental composition of glass and metals and is therefore very helpful in finding specific sources. For example, glass flakes can come from vial delamination, whereas fibers can come from insulation around the plant, or lamination belts used, for example, to make transdermal patches. The presence or absence of elements in glass, including Ba, Ca, K, and Na, can be used to help identify the source. LIBS can identify metal composition as iron, aluminum, copper, or steel and therefore, the source from the processing or cleaning equipment, or from the plant environment can be determined. LIBS spectra of particles can be compared to samples of possible sources for better identification of the specific source. Steps that are taken to avoid the problems in the future would depend on the source. If it is the plant environment, then areas can be cleaned better or the process equipment can be isolated in clean areas. If it is the equipment itself, steps can include preventive maintenance of more frequently replacing parts that wear, or specifying better quality parts. If the source is processing equipment, product-equipment interactions could be the source, and processing parts that will not interact with the product can be selected.
Which Analytical Technique to Use
It is important to know the advantages and limitations of analytical techniques so that the right ones can be used in the right order, and the data will not be misinterpreted. Raman is especially sensitive to chemical bonds with large electron clouds, while IR is sensitive to materials with strong dipoles, thus Raman and IR of the same sample can detect different functional groups to different degrees. For example, Raman can detect cellulose, but in a blue cellulose fiber, the blue dye with aromatic functional groups may give a much stronger spectrum, and the cellulose may not be easily detected. IR has much greater sensitivity to the OH groups in the cellulose, and less sensitivity to the dye with weak dipoles. So for a blue fiber, the cellulose bands dominate the IR spectrum (Figure 5).
Raman can be used to complement the LIBS elemental data because it can identify inorganic complexes and then help determine whether the elements are from pure metals or complexes.8 LIBS spectra destroy about a 10-μm area of a particle, so it would be better to use a non-destructive technique first.
LIBRARIES
Hit Quality Index
Spectra are taken of unknown materials and then typically compared to library spectra for classification and identification of the chemical functional groups present, and where possible, specific identification of the unknown. “Spectral searching is intended as a screening method to assist the analyst, and is not an absolute identification technique, and hence, not intended to replace an expert in infrared spectroscopy and should not be used without suitable training.”9 Spectral searching is done by spectral matching algorithms, which are chosen based on the quality of the spectrum, including noise and baseline, as well as whether an exact match or just chemical identification are needed. Many algorithms work well and can include absolute value, correlation, Euclidean distance, first derivative, and least squares. The result of spectra searching is usually a numerical “match value” or “hit quality index (HQI).” These are numbers derived from a mathematical comparison of two spectra.10 These values are not measures of the material purity, but are simply measures of correlation between the library reference spectra and the unknown spectrum.10 Thus, these values often have less meaning than is commonly attributed to them. Correlation techniques do not provide any information about the probability that the match is valid, are highly dependent on the library content, including mis-named spectra and spectra of contaminated samples, and misidentification can occur between similar materials.11
The best libraries are made with specific known materials from the plant and process that are taken with the same instrument, the same techniques of sample presentation to the instrument, and the same methods of analysis that will be used for unknown material analyses.10
CONCLUSIONS
Particles from vials, syringes, stoppers, plungers, IV bags, process equipment, and filters were counted and identified. This provided information about sources, including wipes, personal protective equipment, glass, and general contamination due to unclean environment. Once the sources are determined, methods can be instituted to prevent contamination, improving product quality.
In order to implement proper analysis, it is important to understand the qualities of the method. For example, in situ analysis of particles in liquids can be complicated by bubbles and surrounding materials. Filtering samples onto appropriate substrates, such as gold-coated filters, was shown to be an excellent method for both counting and identifying particles and was an appropriate substrate for Raman, IR, and LIBS identification. Filtering avoids difficult and time-consuming manual particle isolation.
Understanding which spectroscopic identification technique to use is very important. Often, more than one technique is needed for accurate results. LIBS was shown to be useful for glass and metal identification, Raman was shown to be useful for organics and dyes, and IR was shown to be useful for material with strong dipoles. It was also shown that two techniques provided complementary information that gave more accurate identification.
Because libraries are essential for spectroscopic identification, it is important to understand that the HQI often has less meaning than expected and is highly dependent on library content and quality, as well as the method of analysis. Library quality is best if developed on the same instrument with the same analysis method. The advantages and disadvantages of different analysis methods relative to small particle identification were discussed.
ACKNOWLEDGEMENTS
The authors would like to acknowledge the work of Lin Bui and Justyna Cherris for their help in analyzing the samples.
REFERENCES
1. Barber, TA. Control of particulate Matter Contamination in Healthcare Manufacturing, Boca Ratan, FL, CRC Press;2000.
2. Langille SE. Particulate matter in injectable drug products. PDA J Pharmaceut Sci Tech. 2013;67:3:86-200.
3. Bukofzer S, Ayres J, Chavez A, et al. Industry perspective on the medical risk of visible particles in injectable drug Products, PDA J Pharm Sci and Tech 2015;69:123-139.
4. United States Pharmacopeia (USP) General Chapter <1790>, “Visual Inspection of Injections.”
5. DiGrado CJ. Panel discussion: liquid-borne particle counting in the pharmaceutical industry. III. Method evaluation. Bull Parenteral Drug Assoc. 1970;24:62-67.
6. United States Patent No. 7,016,034. Holz L, Valet O, Lankers, M. March 21, 2006.
7. Curry W, Conway S, Goodfield C, Miller K, Mueller RL, Polini E. Reducing the risk of contamination of sterile parenteral products via ready-to-use closure components. AAPS PharmSciTech. 2010;11(4):1572-1579.
8. Shearer GL. Contaminant identification in pharmaceutical products. The Microscope. 2003;51(1):3-10.
9. ASTM E2310 – 04(2009) Standard Guide for Use of Spectral Searching by Curve Matching Algorithms With Data Recorded Using Mid-Infrared Spectroscopy.
10. Smith BC. Infrared Spectral Interpretation: A Systematic Approach. CRC Press, 1998.
11. Bakeev KA, Chimenti RV. Pros and cons of using correlation versus multivariate algorithms for material identification via handheld spectroscopy. Eur Pharmaceut Rev. 2013.http://www.europeanpharmaceuticalreview.com/wp-content/uploads/Bakeev-Web-Article_proof.pdf.
To view this issue and all back issues online, please visit www.drug-dev.com.
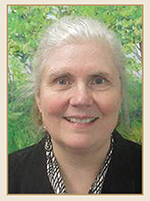
Dr. Kathryn A. Lee is the Lab Head for rap.ID Inc. with experience in pharmaceutical, consumer product, and chemical companies; establishing cGMP-compliant innovative spectroscopic techniques for quality improvement for products and processes. She has held several leadership positions in the Society for Applied Spectroscopy, developed an international course, Practical Problem-Solving in Chemical Analysis, and earned her PhD in Organic Chemistry.
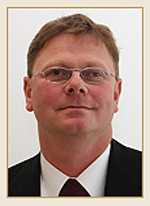
Dr. Markus Lankers is President and Co-founder of rap-ID Particle Systems GmbH that develops and manufactures instruments for rapid particle identification and siliconization measurements. He researches and develops solutions for particulate analysis. He previously worked with Schering AG. He helped to establish the Visual Inspection of Parenterals Interest Group and serves as program co-chair for conferences on Visual Inspection of Parenterals in Europe and USA. He earned his PhD from Würzburg University.
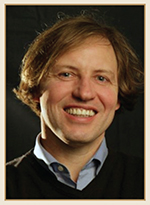
Dr. Oliver Valet is Vice President and Co-founder of rap.ID Particle Systems GmbH, responsible for product development. He has more than 15 years of experience in industrial chemical analysis of particles. As an active member of the Respiratory Drug Delivery, the Royal Chemical Society, Parenteral Drug Association, American Association of Pharmaceutical Scientists, and the Apotekerverband, he has published his work continuously and presented in various conferences. He earned his PhD in Physical Chemistry from Freie Universität Berlin.
Total Page Views: 12692