Issue:March 2015
STANDARDIZATION TECHNOLOGY - Innovative Temperature Standardization Technology Supports Cell Therapy Clinical Trials
INTRODUCTION
Cell-based therapies are primed to transform biological medicinal products in the pharmaceutical industry. The past 2 decades have seen biologics pave the way for a broad range of new treatments, from small molecules to synthetic hormones and antibody-based drugs. Now the use of human and microbial cells as therapeutic entities is challenging the very concept of what constitutes a drug product. There are currently more than 300 cell therapies in late-stage development, and almost 40 more already in commercial distribution.1,2 With this field burgeoning so rapidly, regulatory agencies are striving to introduce new guidelines for manufacturing, storing, and transporting cell therapy drug products.
Cells have therapeutic capabilities distinct from previous classes of biologics. They are naturally programmed to perform therapeutic tasks, such as when T-cells act to mediate or regulate immune response. They can be used to provide new, functional tissue to replace tissue lost to damage or disease. Their method of action is inherently more complex than that of a small biologic because a cell can sense changes in its environment, and mediate its response in a tissue-specific or cell-type-specific manner. Cells are capable of more than one function, including activating diverse signaling pathways, expressing a variety of peptides and proteins, and even migrating to different sites in the body.
NEED FOR STANDARDIZATION
The promise of cell-based therapies is evident in the profusion of drug discovery research dedicated to unlocking its potential. The very complexity that gives cells such promise, however, has led to regulatory concerns about safety and efficacy. Cells are fragile and highly sensitive to even minute changes in their environment. Without proper handling, cells are capable of genetic drift and transformation. This is evident when over-passaged cells no longer express biomarkers specific to their parental cell type, or when stem cells mature into committed progenitor cells that may have lost most or all of their pluripotency. In order to obtain FDA approval, small molecule therapeutics are subject to standards of due diligence. These same standards must be applied to cell therapy, albeit in a manner appropriate to this new technology.
Cell, tissue, and gene therapy products are so functionally and biologically diverse that the US FDA acknowledges “unique scientific challenges in terms of regulatory review.” In 2007, the European Medicines Agency saw fit to establish an entirely new set of guidelines based on these treatments, which are classified as “advanced therapy medical products.”3,4 To ensure quality products, the natural variability of cell therapies must be met with meticulous standards of reproducibility. The key to reproducibility is standardization of common laboratory or manufacturing practices, in particular, protocols for cell culture, cryopreservation, and storage. Cellular viability is highly dependent on cold chain management during transport, handling, and storage. BioCision, a private company founded in 2007 in Larkspur, CA, has developed unique proprietary temperature standardization technology that is practical for all stages of cell processing and handling.5 This technology is supporting a Phase IIb clinical trial conducted by TxCell, a French company specializing in cell-based therapies for chronic inflammatory diseases. The trial is investigating the efficacy of Ovasave®, an immunotherapy treatment for patients with moderate-to-severe refractory Crohn’s disease.
REGULATORY T-CELL THERAPY
Regulatory T-cells (Tregs) are a subpopulation of T-cells that modulate immune response through interaction with other lymphocytes at the molecular level. Tregs can inhibit immune cell activation and unwanted inflammatory response to foreign antigens that are normally tolerated, such as dietary, bacterial flora, or inhaled antigens. They can also suppress pathological immune responses, like those involved in autoimmune disorders. Ovasave is an autologous, antigen-specific Treg (Ag-Treg) that targets the diet antigen ovalbumin. It has been shown to be a well-tolerated, effective treatment for Crohn’s disease in Phase I/IIa clinical trials.6
Tregs are extremely sensitive to variations in temperature, which can affect viability, recovery, and functionality.7 Consequently, temperature control is a critical aspect of Treg sample handling. The cryopreservation process figures large in any cell-related study, and is of particular importance in the Ovasave study because the same blood sample is used to make multiple doses over several years of patient treatment. Successful cryopreservation depends on a tightly controlled rate of temperature decrease during freezing, specifically -1°C/min.8
DEVELOPING A NEW CELL-FREEZING CONTAINER
Current methods of cryopreservation include freezing cells in isopropanol-filled devices, which necessitates long equilibration times and can introduce variability depending on vial position, or the use of programmable freezers. The latter are documented to have highly reproducible freeze rates, but are also costly, difficult to maintain, susceptible to malfunction, and require a large footprint in terms of space and energy use.
BioCision’s CoolCell® cell-freezing containers (Figure 1) are passive freezing devices that deliver a consistent -1°C/minute freeze rate to all cryogenic vials when placed in a -80°C freezer. This is achieved through the unique design of the CoolCell container, which is made of a highly insulative closed-cell polyethylene foam material. Cryogenic vials are placed in a radially symmetric manner around a solid alloy thermal core that ensures identical heat removal profiles for each vial. This combination of insulative and thermally conductive materials ensures high thermal control and reproducibility. The simple, single-block base design makes the CoolCell container extremely durable and maintenance free, while a small footprint permits use across multiple study sites. CoolCell cell freezing containers may be used with cryogenic vials or closed-system injectable ampules or vials.
The device has been used successfully in the preservation, storage, and recovery of human stem cells, and is recommended for use by ATCC®, the world’s leading provider of authenticated biological material, in their current stem cell handling guide. Consequently, TxCell decided to investigate whether to incorporate the use of CoolCell in its ongoing Ovasave clinical trials, in place of programmable freezers.9
CLINICAL TRIAL METHODS
In order to isolate clinical-grade Ova-Treg products, peripheral blood mononuclear cells (PBMCs) were first collected from healthy donors, isolated via a Ficoll gradient, and then cultured in the presence of ovalbumin to expand ova-specific T-cells. Following a week in culture, T-cells were cloned and selected based on ovalbumin-specific IL-10 production. Both PBMCs and Ova-Treg cells were aliquoted into 2-ml closed-system glass ampules containing appropriate cryopreservation media in preparation for freezing, and then frozen at varying dosage. Cell viability was determined before and after freezing, via propidium iodide staining combined with flow cytometry.
In accordance with European Union Good Manufacturing Practice (GMP) cleanroom guidelines, CoolCell cell-freezing containers must be sanitized with two surface cleaning and disinfectant solutions.10 The effectiveness of the cleaning procedure was assessed by measuring the particle emission profile on a particle counter, and measuring microbial counts on gelose plates. Cells were then frozen using the CoolCell cell-freezing container placed in a -80°C freezer.
STUDY RESULTS
The researchers first tested the performance of the CoolCell cell-freezing container in five back-to-back runs. Because the device requires no isopropanol or other fluids, it is possible to maintain a mere 5- to 10-minute wait period between runs. The five consecutive runs yielded tightly reproducible freezing profiles, with each of the 12 cryovials having a freezing rate of -1°C/min (Figure 2). This rate is considered optimal for post-thaw cell viability, and is the same as that obtained with a programmable freezer.
Cell viability was assessed next for both PBMCs and Ova-Treg cells. Prefreezing cell viability for PBMCs was consistently over 95%. Viability was assayed for PBMCs frozen either in a controlled-rate programmable freezer, or using the CoolCell cell-freezing container. In both protocols, cells were frozen and maintained at -80°C, then stored at -150°C for 5 days. Postthawing, PBMC viability was analyzed on a flow cytometer using propidium iodide; a stain which is excluded from living cells. Analysis showed there was no significant difference in viability rates between cells frozen using a programmable freezer or those frozen in a CoolCell container (Figure 3).
Researchers then measured viability using a similar experimental set up in target cells, the Ova-Tregs that are used for the Ovasave clinical trials. FDA guidelines require cell viabilities for cell therapies to be over 70%.11 In this study, pre-freezing viability for all cells was consistently over 90%. Once again, no significant difference in post-thaw cell viability was observed between controlled-rate freezers (91.7% ± 4.0%) and cells frozen using the CoolCell container (91.7% ± 3.7%) (Figure 4). Similarly, there was no significant difference in cell yield using either the CoolCell cell-freezing container or a programmable freezer.
Because all ex-vivo cell handling and cryopreservation procedures for cell therapy manufacturing must be performed in adherence to EU GMP cleanroom regulations, researchers also needed to assess whether the CoolCell cell-freezing container met these regulatory requirements.9 After subjecting CoolCell containers to standard cleanroom procedures, particle-release profiles and microbial contamination assays were performed. Three different CoolCell cell-freezing containers were tested three different times. In each case, CoolCell container profiles were well below the acceptable particle-release level (Figure 5). Decontamination procedures were also effective in containing microbial contamination to a level suitable for a class B cleanroom. In light of the results obtained in these proof-of-principle studies, the researchers elected to incorporate the CoolCell cell-freezing container into their clinical trials going forward.
IMPACT ON FUTURE CELL THERAPY CLINICAL TRIALS
The results demonstrate that the CoolCell cell-freezing container is a suitable alternative to a controlled-rate freezer for clinical studies. The TxCell Phase IIb clinical trial is of particular interest in that it resulted in the decision to switch to the CoolCell container despite the fact that controlled-rate programmable freezers had been used in the primary stages of the trials. This implies that the new device represents not simply an alternative to other freezing methods, but in fact is preferable in that it offers several very significant advantages in comparison to conventional methods.
One of the most obvious drawbacks with conventional methods, especially for multi-site clinical trials, is the high cost of a dedicated controlled-rate freezer. This will affect preclinical development as well as clinical, and the cost consideration will only be magnified going forward because many successful drug candidates will have to be scaled up for commercial development and manufacturing. The CoolCell cell-freezing container is designed for use with a -80°C freezer, which is standard equipment even in academic labs. Furthermore, due to the small size and portability of the CoolCell container, the freezer it resides in need not be dedicated to cell freezing, an important point for most researchers in the R&D stages of development, when the cells in question generally represent one of many drug candidates. Cost of maintenance must also be considered. Set up and maintenance of an instrument as large and sophisticated as a programmable freezer isn’t trivial, and precious samples could be lost if a malfunction were to occur. The simple one-piece design of the CoolCell cell-freezing container requires no maintenance, and virtually no training, giving the device the additional advantage of eliminating variability in how it is employed from researcher to researcher, or from site to site.
The use of a portable, passive freezing device will also have a positive impact on scalability, being easier and less expensive to employ at multiple clinical sites. For future scale up of Ovasave manufacturing, larger capacity CoolCell units may be used, or multiple devices might be used in parallel. The CoolCell container can be used in combination with other BioCision temperature standardization products. TxCell, for example, is also deploying the CoolRack modules and CoolBox ice-free cooling systems to minimize temperature variation and lower the risk of contamination during its cell handling and preparation stage.
Cell therapy carries an intrinsic variability, for the simple reason that each person is different. Not every blood sample drawn from a healthy donor will contain an identical number of target cells with the same potency from cell to cell. Not every patient will respond to therapy in the same manner. For cell therapies to succeed in the clinic, standardized protocols for cell culture, handling, and freezing should be instituted to guarantee the highest possible standards in reproducibility. We need to identify those steps at which variation can be eliminated. Years of intense cell therapy research have made it clear that even a slight variation in temperature at any point during sample handling can potentially affect the efficacy or potency of the final therapeutic product.
Cryopreservation protocols are of singular importance to the entire drug development process, because while fail-safes, such as screening assays, may be employed at earlier steps, once a cellular product is frozen for cryopreservation, its next intended use is for patient treatment. As is the case for the TxCell Ovasave clinical trial, a single blood sample may represent years of patient treatment once target cells are expanded. Steps taken to ensure maximum quality and reproducibility of cell samples will no doubt carry over into non-clinical areas as well. Basic biomedical research, preclinical studies, and biobanking all stand to gain from standardization of sample handling. The development and use of temperature standardization technologies that is cost-effective, maintenance free, and easy to use is an important step in the right direction.
To view this issue and all back issues online, please visit www.drug-dev.com.
REFERENCES
1. Buckler, RL. Cellular therapy wave finally cresting. Genetic Engineering News. 2012;32(19).
2. Buckler, RL. Rise of cell-based immunotherapy. Genetic Engineering News. 2013;33(20).
3. US Food and Drug Administration. Guidance for industry: preclinical assessment of investigational cellular and gene therapy products. 2013.
4. European Medicines Agency. Regulation (EC) No 1394/2007 of the European Parliament and of the Council. 2007.
5. Shu Z, Kang X, Chen H, Zhou X, Purtleman J, et al. Development of a reliable, low-cost controlled cooling rate instrument for the cryopreservation of hematopoietic cells. Cytotherapy. 2010;12(2):161-169.
6. Desreumaux P, Foussat A, Allez M, Beaugerie L, Hébuterne X, et al. Safety and efficacy of antigen-specific regulatory T cell therapy for patients with refractory Crohn’s disease. Gastroenterology. 2012;143(5):1207-1217.
7. Golab K, Leveson-Gower D, Wang X, Grzanka J, et al. Challenges in cryopreservation of regulatory T cells (Tregs) for clinical therapeutic applications. International Immunopharmacology. 2013;16(3): 371-375.
8. Yokoyama WM, Thompson ML, Ehrhardt RO. Cryopreservation and thawing of cells. Current Protocols in Immunology. 2012. Appendix 3:3G.
9. Foussat A, Rietze R, Pottier E, Schryver B, et al. Effective cryopreservation and recovery of human regulatory T cells. BioProcess International. 2014;12(S33):34-38.
10. EU Guidelines to good manufacturing practice: Medicinal products for human and veterinary use annex 1: manufacture of sterile medicinal product. 2009;4.
11. US Food and Drug Administration. Guidance for FDA reviewers and sponsors: Content and review of chemistry, manufacturing, and control (CMC) information for somatic cell therapy investigational new drug applications (INDs). 2008.
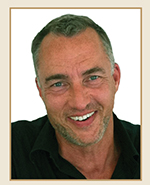
Dr. Rolf Ehrhardt is the President and CEO of BioCision. Prior to this, he was responsible for the early (Phase 1a) clinical development of a novel HCV protease inhibitor at Intermune. Before that, he was the Vice President of Preclinical Development at Corgentech and Founding President of BioSeek. Dr. Ehrhardt was a Deutsche Forschungsgemeinschaft (DFG: German Research Foundation) and Fogarty Fellow at the NIH, where his focus was on mucosal immunology and inflammatory bowel disease. Dr. Ehrhardt earned his medical and doctoral degrees with distinction from the Technical University of Munich, Germany.
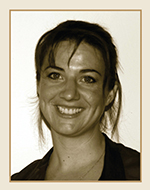
Dr. Maria Thompson is the VP Scientific Affairs with BioCision. She has a background in molecular genetics and over 16 years of experience in pharmaceutical and diagnostics research and development. She has held a variety of roles, including Head of Genome Wide Screening for type 2 diabetes, Six Sigma Black Belt, Principal Consultant, and Head of Scientific Affairs, as well as running APEX Think, a private scientific writing and marketing consultancy. Dr. Thompson earned her BSc in Genetics and her PhD in Molecular and Cellular Biochemistry from the Royal London School of Medicine, UK.
Total Page Views: 3425