Issue:March 2018
NASAL DELIVERY - A Promising Route of Drug Delivery to the Brain: Scientific Considerations
ABSTRACT
One of the factors that limit the ability of most of the drugs to treat Central Nervous System (CNS) disorders is related to the extent of drug that is able to cross the Blood Brain Barrier (BBB). The endothelial cells of the blood capillaries to the brain include tight junctions that act as a barrier to most drugs and inhibit the ability of drugs and solutes to cross this barrier. The BBB effectively restricts the transfer of hydrophilic compounds from the vascular compartment to the brain tissue. In contrast to the other tissues, no bulk flow occurs across the capillary walls due to tight junctions between the cells. Throughout the past few decades, there has been a number of innovative drug delivery approaches that may overcome the challenges associated with drugs to cross the BBB.
One such approach entails the delivery of drugs via the nasal route. There is growing scientific support that delivery of drugs via the nasal route may result in higher concentrations of drugs that can cross the BBB. However, this approach has significant limitations that require a careful consideration of the physico-chemical and pharmacological properties of the drug, it’s potential CNS toxicity, as well as the dose and delivery vehicles that may be used. The final assessment after performing the review on this subject indicates there are significant differences in the nasal anatomy and physiology of different animal species and humans, which makes it very difficult to obtain a direct correlation between them. The published experimental data in scientific journals does support that different formulation approaches using mucoadhesive compounds, absorption enhancers, and specialized reagents can increase the efficiency of drug delivery to the brain via the nasal route. Further experiments are needed to establish a robust correlation between the properties of the compound being investigated, the physiology of the nasal cavity, and the impact of specialized drug delivery techniques that are known to influence drug delivery to the brain via the nasal route of administration.
INTRODUCTION & BACKGROUND
Drugs are delivered to the systemic circulation via several routes, such as oral, parenteral (intravenous, intramuscular), and in most cases, drugs administered via these routes encounter acidic or enzymatic degradation and may undergo excessive first-pass effect (hepatic metabolism) following administration. Due to these factors, effective doses of drugs sometimes may not reach the systemic circulation, resulting in ineffective treatment. It is therefore required to explore either alternate routes or specialized delivery technologies that can result in improved and effective drug delivery options. The nasal route of drug delivery is one such alternate route that provides access to highly vascularized mucosa, which can be exploited as an interesting site for local drug delivery, systemic drug delivery, and targeted drug delivery (CNS).
The anatomy and physiology of the olfactory region is such that it can provide a direct path to the CNS, resulting in higher concentration of drug in different regions of the brain. The additional benefit of this region is that it provides both intracellular and extracellular drug transport pathways to the CNS. In order for a certain drug to get transported via one of these two pathways, it is also dependent on other factors that are related to the physico-chemical properties of the drug and specific receptors present on the olfactory neurons.
Nasal applications of topical decongestants or anti-inflammatory drugs are the most popular topical nasal drug deliveries. For some compounds, delivery of drugs via the nasal route provides direct access to systemic absorption. Absorption of drug via the nasal cavity can be described as diffusion of drug into system circulation via the nasal mucosa. Mucosal absorption via the nasal cavity usually follows: drug release, penetration (entry into a layer), permeation (transition of a layer), and absorption (uptake into the vascular system). Assuming the existence of an olfactory-pathway to the brain in humans, it remains an area to explore and understand as to what extent it contributes to central nervous availability of drugs administered via the nasal route.
THE NASAL ANATOMY
The nasal septum divides the human nose into two equal symmetrical halves. The posterior part of the nasal cavity is called the nasopharynx, and each symmetrical half opens to the environment. Both halves of the nasal cavity consist of the following four regions:1,2
Vestibule: is not very highly vascularized and permeability of the drugs via this region is very poor.
Atrium: vascularization in this part of the nasal cavity is low, which results in moderate permeability of drugs.
Respiratory Region: this part of the nasal cavity is highly vascularized and therefore the permeability of drugs from this region is good.
Olfactory Region: is highly vascularized, which results in high permeability of drug. This region is also reported as a potential site for nose-brain transport of drugs.
BARRIERS TO DRUG TRANSPORT FROM NOSE TO BRAIN
Physico-Chemical Properties of the Drug
Molecular weight, lipophilicity, and degree of dissociation are the primary properties of the drug that dictate to what rate and extent drugs will transport from the nasal mucosa to the brain.
Regarding relative molecular weight, there have been studies in which the effect of molecular weight was studied, and it was observed that as the molecular weight of drug administered via the nasal route increased, the concentration of drug in the brain decreased. Different molecular weight of fluorescent-labelled dextrans FD4 (4400 Da), FD20 (18900 Da), and FD40 (40500 Da) were administered via the nasal and intravenous routes. The concentration of drug in the cerebrospinal fluid (CF) were evaluated following iv administration, and it was found there were no fluorescent labelled dextrans detected in the CSF. Although FD4, FD20, and FD40 were detected in the CSF following nasal administration, the concentration decreased as the molecular weight of the dextrans increased. Most small molecular weight (<400 Da) drug molecules get absorbed easily and are good candidates for transport to the brain via the nasal mucosa.3 Molecular weights higher than 1000 Da show poor ability to get absorbed in to the brain via the nasal mucosa. Large molecules, such as peptides and proteins, have also been evaluated for their capability of getting absorbed via the nasal cavity.3
Regarding lipophilicity, lipophilic drugs are known to show high absorption via the nasal mucosa. This nasal epithelium is known to behave as a lipid sieve, which makes the lipophilic drugs penetrate the nasal mucosa. It is also observed that there exists a linear correlation between the drug’s oil-water distribution coefficient and its absorption rate constant.4 Lipophilic drugs, such as sulfonamides, have been tested in animal models in which the drug concentration in the CSF increased as a result of direct nose-to-brain transport following nasal administration. Lipophilic drugs, such as alprenolol and propranolol, were well absorbed from the nasal mucosa when compared with the hydrophilic drug metoprolol.5
Regarding degree of dissociation, the drug concentration in the CF is inversely proportion to the degree of dissociation. It is therefore important to understand that the degree of ionization of a drug that is administered via the nasal route can affect the ability of drug to get absorbed in the nasal mucosa and its concentrations in the CSF. Diltiazem HCl and paracetamol have been used as model drugs to study the physio-chemical properties of the drug in relation with nasal absorption. The results of this study concluded that there exists a quantitative relationship between the partition coefficient and the nasal absorption constant.4
Drug Concentration, Dose & Volume of Administration
The concentration of drug, dose, and volume of dose administered are all important factors that can affect nasal drug delivery to the brain. Nasal drug absorption increases as the concentration of drug increases at the site of administration. This phenomenon is more prominent with drugs that are absorbed by passive diffusion as a primary mechanism of drug absorption. Higher concentrations of drug administered in high volume can negatively impact the absorption of the drug due to local adverse effects and in some cases, results in damaging the nasal mucosa. This is why it becomes important to realize that the nasal cavity has limited capacity and therefore the dosage for nasal administration must be relatively low (25 to 200 μl).6
Mucociliary Clearance
One of the important functions of the nasal cavity is the removal of dust, allergens, and bacteria as part of normal physiological function. For drugs to maintain the desired concentration and delivered volume following nasal administration so that the therapeutic dose can get absorbed, it is important the drugs display prolonged residence time within the nasal cavity. The deposition of drug in the nasal mucosa can be achieved by different formulation approaches and by maintaining the pH of the nasal formulation in the range of pH 4.5 to 6.5.7 Different dosage forms have been used to increase the residence time in the nasal cavity. These include gelatin, emulsions, ointments, liposomes, microspheres, and nanoparticles prepared using ion exchange resin methods. Bioadhesive preparations, starch microspheres, and chitosan-based formulations have been extensively studied, which resulted in improved bioadhesive properties and increased residence time on the nasal epithelial surface.8,9
Presence of Enzymatic Activity
The presence of enzymes in the nasal cavity can form an enzyme barrier that is known to affect the stability of the drug in the nasal cavity. Proteins and peptides are prone to degradation by proteases and amino-peptidase within the nasal cavity. Although it is not exact as the first-pass effect that drugs undergo following oral administration, the enzymatic activity in the nasal cavity can result in decreased therapeutic effects. The presence of P450 enzymes are much higher in the nasal mucosa when compared to the respiratory mucosa.10
Difference in Animal Species
The nasal mucosa and its physiology is very different from one species to another. Surface area of the olfactory mucosa varies with different animals. Rats and mice have been widely used as experimental animals. The olfactory area in rats is more than 50% of the entire surface area of the nasal cavity as compared to humans in which the olfactory region is only 3% to 5% of the entire nasal cavity. It is therefore very important to take into account the anatomical and histological differences (Table 1) when extrapolating findings from animal experiments to humans.11-13
RECOMMENDATIONS TO OVERCOME BARRIERS TO DRUG TRANSPORT FROM NOSE TO BRAIN
There have been a number of novel approaches evaluated in animal models to overcome the barriers to nose-to-brain delivery of drugs via the nasal route. The efforts have been concentrated toward increasing the residence time in the nasal mucosa and modifying the physico-chemical properties of the drug using functional excipients and innovative drug delivery technologies. A few examples of these innovative technologies include a combination of mucoadhesive polymers, absorption enhancers, and drug delivery devices aimed for precise delivery of drug within the nasal cavity.
Prodrug Approach
As previously discussed, the physicochemical properties of drugs, such as the molecular weight and lipophilicity, are critical parameters that have the most influence on drug delivery to the brain via the nasal epithelium. A prodrug strategy can help in modifying these properties in such a manner that the rate and extent of drug absorption increases in the nasal cavity. Experimental studies both in vivo and ex vivo have shown that rapid and complete absorption of drug can be attributed to the degree of lipophilicity and smaller molecular weight of the test compound. Several water-soluble alkyl ester prodrugs of Ldopa were administered to rats via the nasal route, and it was observed that the concentration of butyl ester prodrug of Ldopa was significantly higher in the CNS of rats as compared to parent drug.14 While this approach has proven to work in many small molecules, this strategy presents some challenges for large molecules, such as proteins and other biologics. It has been difficult to increase the lipophilicity of proteins as there can be significant impact on the spatial structure of the protein, resulting in diminished biological activity.14
Innovative Formulation Approach
Maintaining high drug concentration for passive diffusion on the nasal epithelium is important, and in order to achieve this, precise drug deposition and extended residence time must be optimized. There are several nasal formulations and devices that are designed to overcome these challenges. Experimental design in which N-cyclopentyladenosine (CPA) was formulated with mannitol-lecithin and chitosan hydrochloride microparticles were administered to rats via nasal administration showed higher amount of CPA present in the CNS of rats compared to the free CPA. The chitosan hydrochloride formation resulted in a 10-fold higher amount of CPA in the CSF compared to the mannitollecithin microparticles formulation.16
Absorption Enhancers & Enzyme Inhibitors
Drugs that are highly lipophilic in nature and also have a very low molecular weight might not need a specialized formulation approach, including use of absorption enhancers. Absorption enhancers can be used in cases where the drug exhibits poor membrane permeability, has large molecular size, and is susceptible to enzymatic degradation by aminopeptides.17,19,20 Drugs that are formulated using absorption enhancers may impart the following properties that will result in increased drug bioavailability following nasal administration:
– Improve the solubility of the drug
– Reduce the surface tension of the mucus
– Decrease the enzyme activity which may keep the drug in its stable form
Nasal Drug Delivery Devices
Drug delivery devices have been found to play an important role in ensuring that the entire drug is delivered to the target site in the nasal cavity. It is difficult to precisely deliver the drug to the olfactory region of the human nasal cavity as this region is found high up in the nasal cavity, above the superior conchae. This area is exposed to a very low volume of the air that penetrates the nasal cavity and can result in lower doses of the drug reaching the olfactory region. Some of the novel proprietary devices that have shown significant differences following administering the drug via the nasal route are shown in Table 2.18-21
Although the initial proof-of-concept studies using these novel nasal drug delivery devices does show promising results, they still need to be further tested using different types of molecules intended to be delivered to the CNS/brain via the nasal cavity/route of drug administration.
CONCLUSION
Administration of drugs via the nasal route is probably one of the most non-invasive methods of bypassing the BBB for delivering drugs targeted for CNS disorders. After reviewing the clinical experiments published in this area of drug delivery, it is evident that formulation design, altering the physico-chemical properties of the drug, addition of absorption enhancers and mucoadhesive polymers did result in higher bioavailability of drugs in animal models via the nasal route when compared to parenteral administration of the same drug. Figure 1 shows a combination of ideal parameters that will maximize nose-to-brain drug delivery.
Because the ultimate goal of performing studies in animal models is to collect data and parameters that can be translated to human studies, there still exists a gap in establishing meaningful correlations. The differences in the anatomy and physiology of the animal nose and human nose are very significant and makes it difficult to correlate the outcome of the studies. In addition, the correlation between the amounts of dose delivered via the nasal route versus the amount of dose bioavailable in the CNS of animal species does not exist. This is due to the reason that high volumes of doses are used in the clinical studies using animal models, and these doses are practically not possible for human administration.
ACKNOWLEDGEMENTS
The author would like to thank Drs. Pankaj Desai, William Siebel, and Giovanni Pauletti of the James L Winkle College of Pharmacy, University of Cincinnati, for their guidance and support.
REFERENCES
1. Temsamani J. Delivering drugs to the brain—beating the blood brain barrier. Eur Biopharm Rev Autumn. 2002:72-75.
2. Schwartz MW, Sipols A, Kahn SE, Lattemann DF, et al. Kinetics and specificity of insulin uptake from plasma intocerebrospinal fluid. Am J Physiol. 1990:259:E378–E383.
3. Djupesland PG, Skretting A. Nasal deposition and in man: comparison of a bidirectional powder device and a traditional liquid spray pump. J Aerosol Med Pulm Drug Del. 2009;25:280-289.
4. Sakane T, Akizuki M, Yamashita S, et al. The transport of a drug to the cerebrospinal fluid directly from the nasal cavity: the relation to the lipophilicity of the drug. Chem Pharm Bull. 1991;39:2456-2485.
5. Jiang XG, Lu X, Cui JB, et al. Studies on octanol-water partition coefficient and nasal drug absorption. Acta Pharm Sin. 1997;32:458-460.
6. Ross TM, Martinez PM, Renner JC, et al. Intranasal administration of interferon beta bypasses the blood brain barrier to target the central nervous system and cervical lymph nodes: a non-invasive treatment strategy for multiple sclerosis. J Neuroimmunol. 2004;151:66-77.
7. Arora P, Sharma S, Garg S. Permeability issues in nasal drug delivery. Drug Discov Today. 2002;7:967-975.
8. Illum L. Nasal drug delivery compositions containing nicotine. 1999;US5935604.
9. Pavis H, Wicock A, Edgecombe J, et al. Pilot study of nasal morphinechitosan for the relief of breakthrough pain in patients with cancer. J Pain Symptom Manage. 2002;24:598-602.
10. Vyas TK, Shahiwala A, Marathe S, Misra A. Intranasal drug delivery for brain targeting. Curr Drug Deliv. 2005;2:165-175.
11. Mygind N, Dahl R. Anatomy, physiology and function of the nasal cavities in health and disease. Adv Drug Deliv Rev.1998;29(1):3-12.
12. Lochhead JJ, Thorne RG. Intranasal delivery of biologics to the central nervous system. Adv Drug Del Rev. 2012;64:614-628.
13. Gizurarson S. Anatomical and histological factors affecting intranasal drug and vaccine delivery. Curr Drug Deliv. 2012;9:566-582.
14. Dalpiaz A, et al. Brain uptake of a Zidovudine prodrug after nasal administration of solid lipid microparticles. Mol Pharm. 2014;11:1550-1561.
15. Zachary N, Warnken H, Smyth DC, et al. Formulation and device design to increase nose to brain drug delivery. J Drug Delivery Science & Tech. 2016;35:213-222.
16. Johnson NJ, Hanson LR, Frey WH II. Trigeminal pathways deliver a low molecular weight drug from the nose to the brain and orofacial structures. Mol. Pharm. 2010;7:884-893.
17. Wolf DA, et al. Lysosomal enzyme can bypass the blood-brain barrier and reach the CNS following intranasal administration. Mol Genet Metab. 2012;106:131-134.
18. Costantino HR, Illum L, Brandt G, Johnson P, et al. Intranasal delivery: physiochemical and therapeutic aspects. Int J Pharm. 2007;337:1-24.
19. Appu AP, Arun P, Krishnan JKS, et al. Rapid intranasal delivery of chloramphenicol acetyltransferase in the active form to different brain regions as a model for enzyme therapy in the CNS. J Neurosci Methods. 2016;259:129-134.
20. Karasulu E, Yavas A, et al. Permeation studies and histological examination of sheep nasal mucosa following administration of different nasal formulations with or without absorption enhancers. Drug Delivery Technology. 2008;15:219-225.
21. Djupesland PG. Nasal drug delivery devices: characteristics and performance in a clinical perspective – a review. Drug Deliv Transl Res. 2013;3:42-62.
22. Lisbeth I. Intranasal Delivery to the Central Nervous System: Book Chapter
25. (Blood–Brain Barrier in Drug Discovery: Optimizing Brain Exposure of CNS Drugs and Minimizing Brain Side Effects for Peripheral Drugs, First Edition. Edited by Li Di and Edward H. Kerns. © 2015 John Wiley & Sons, Inc. Published 2015 by John Wiley & Sons, Inc.).
To view this issue and all back issues online, please visit www.drug-dev.com
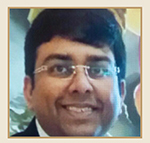
Vinayak Pathak currently serves as Associate Director, Product Development at West-Ward Pharmaceuticals, based in Columbus, OH. He earned his BS in Pharmaceutical Sciences from India and his Master’s in Pharmaceutical Sciences from James L Winkle College of Pharmacy, University of Cincinnati. He also earned his MBA from Columbus State University, GA. He is leading a team of formulation scientists and involved in initial formulation development to pilot GMP mfg. and technology transfer. Previously, he served as G roup Leader, Formulation Development at Accucaps Industries ltd, Canada, where he was responsible to lead a team and develop formulation and manufacturing process for soft gelatin capsules. He also worked at Pharmascience Inc, Montreal Canada, as Senior Scientist, Formulation Development, where he was responsible for formulation development and business support activities for various dosage forms involving solids, liquids, and suspensions. Mr. Pathak’s research focus includes development of immediate-release, controlled-release systems, multiparticulate drug delivery systems, and nasal formulation development.
Total Page Views: 39590