Issue:May 2017
CONTROLLED RELEASE - Leveraging Precision Particle Fabrication® Technology to Create Patient-Friendly Dosage Forms
INTRODUCTION
The rising cost of healthcare and the resultant shift of policies focused on lowering costs and incentivizing better outcomes are driving increased demand for drug delivery solutions that can improve patient compliance and provide economical solutions for special patient populations, such as pediatrics and geriatrics. Controlled-release solutions play a critical role in patient-centric design, offering the ability to reduce the number of administrations required for the patient. The need for controlled release may vary for specific patient populations: getting a child through the school day or helping elderly patients better manage their multiple medication regimes. However, the evidence is clear that reducing the number of administrations is correlated to an increase in medication compliance and therefore improves patient outcomes.1 Palatability of a drug product can also impact compliance, and therefore, taste is also an important consideration when creating patient-friendly products. Unpleasant taste has been documented as one of the biggest barriers to completing treatment in pediatric patients with more than 90% of pediatricians reporting that a drug’s taste and palatability were the biggest barriers to completing treatment.2 The ability to appropriately address both controlled release and palatability in next-generation formulations offers the opportunity to significantly improve patient outcomes. While current approaches do exist to create controlled-release and taste-masked solutions, the ability to do so in a patient-friendly format can be fraught with formulation challenges of its own.
CONTROLLED-RELEASE CHALLENGES
Attaining controlled-release kinetics with tablets is a relatively simple process, as the size and form factor of the dosage form lends to using vigorous coating methods, oftentimes with multiple layers.3,4 Capsules have the advantage of being moulded, extruded, or pressed with gelatin and other dissolution-enhancing excipients in a high-throughput manner, enabling delivery of large doses of medication. Tablets are simply pressed, then coated with subsequent layers of controlled-release components, which makes adaptation of specialized kinetics straightforward.3,4 The key challenge to these techniques is that these multiple coating steps and the larger doses associated with reducing administrations often results in a large tablet or capsule size. This becomes problematic in patients for whom swallowing traditional oral solid dosage forms is a challenge. Data from current tablets and capsules indicates that the average size of a controlled-release dosage form is nearly 1.5 cm in length.5 Physiological studies demonstrate that swallowing becomes difficult when the length of the object being swallowed is greater than half of the esophageal width, which is approximately 2.0 cm for the prototypical adult.6 This means dosage forms over 1 cm in length, smaller than the average modified-release pill, may be too large to be swallowed easily by much of the adult population.
The scarcity of dispersible format oral products sometimes leads caregivers and compounding formularies to use alternative solutions to treat their patients that are not always backed by supporting safety, bioavailability, and stability studies. Tablets are sometimes modified to ease administration challenges. Modifications can include splitting the dosage form or crushing the dosage form and mixing with food or drink. These methods may result in dosing errors and decreased efficacy, and can magnify non-adherence if the active pharmaceutical ingredients (API) is foul-tasting.3,4 The risk associated with altering controlled-release tablets is significant as patients or caregivers may be unaware of the risk of dose dumping that this presents. This risk is highlighted by the annual publication of the “Oral Dosage Forms That Should Not Be Crushed” list published by the Institute for Safe Medication Practices.7-16 Drugs listed in this publication are most commonly included due to their controlled-release characteristics and the risk of dose dumping associated with crushing a controlled-release product; others are selectively included for taste and exposure reasons.
The ability to provide controlled-release kinetics with format-flexible dosage forms is fundamental for serving special populations. When considering just pediatrics and geriatrics, this dosage form issue affects over half of the global population (under 18 and over 65 years of age).3,4,17,18 However, the need extends well beyond these patient populations as there are specific therapies and indications that induce dysphagia, as well as healthy adults that suffer from dysphagia as well.
TASTE-MASKING CHALLENGES
The demand for taste-masked product solutions will be driven primarily by pediatric markets; however, in the case of very bitter-tasting drugs, adults and children alike can benefit from palatable solutions. While many solid oral dosage forms have techniques for masking or encapsulating bitter tastes, these methods are ineffective for many children (and some adults) because they often cannot or will not swallow pills or tablets.2
For pharmaceutics with extremely bitter taste, coating techniques can be used; however, some can result in an unpleasant mouth feel due to surface roughness. It has been estimated that nearly half of patients with organoleptic sensitivities are disinclined to take their medicine, with the majority of those reporting bad taste as a large contributor to non-compliance.12 Artificial sweeteners and flavors are often unable to conceal the extremely unpleasant taste of many APIs in liquid formulations.7-9 What is worse, efforts to mask foul taste using coatings or microencapsulation sometimes create poorly controlled, polydisperse particle diameters that result in a gritty consistency. In a perfect scenario, a dosage form would accomplish taste-masking with negligible texture, while maintaining controlled-release properties.
LIQUIDS OFFER FORMAT FLEXIBILITY BUT GENERALLY NOT CONTROLLED RELEASE
The need for format flexibility has generally been attributed to pediatric patients due to their inability to swallow pills. However, this need extends well beyond pediatrics and is better characterized when including the need for dose titration as well. Patients with differing ages, weights, body surface areas, and metabolic profiles may require atypical dosing considerations.13 Additionally, some therapeutics require titration of dose up or down when initiating or ending a prescribed course of treatment or when optimizing for best outcome. When prescribing medications in older adults, the old maxim “start low and go slow” typically applies to reduce incidence of adverse events, so titration is a key attribute of a product utilized by this population. These examples demonstrate the need for format flexibility not only for those that are unable to swallow a pill, but also for those that require dose titration.
When large oral dosage forms pres-ent administration difficulties or titration is necessary, liquid formats, in general, succeed in providing an acceptable solution. The advantages beyond ease of dosing, however, are limited in traditional syrups with solubilized API. Liquid formats are usually not extended release, have nominal taste-masking, and can contain API particles, or encapsulated-API particles prone to aggregation and settling if not reconstituted or shaken properly prior to administration, which have resulted in risks to patient safety.7,12,13,19-21 Due to the large size of modified-release tablets, the foul taste of traditional liquids, and the lack of controlled-release options for APIs in nearly all marketed drugs,22,23 many pharmaceutical and contract development manufacturing organizations (CDMOs) are focusing efforts on modified-release powder formats, which combine the stability of traditional solid oral dosage forms with dose titration advantages of liquids.
POWDERS: THE OTHER SOLID DOSAGE FORM
While the evolution of technology utilized in tablets, capsules, and pills over the past century is remarkable, the pace at which powder dosage forms are leveraging their share of the market in the past 2 decades is equally impressive. The most recent advances in controlled-release powder technology offer patient-centric solutions that have solid oral dosage form complexity and all the advantages of liquid format flexibility. However, the processes currently utilized to create controlled-release powders can involve complex processing, inclusion of materials such as polystyrene, broad particle size distributions resulting in unpredictable release kinetics, or particle size limitations that create unsatisfactory mouth feel; nearly all methods require multiple coating steps following initial particle fabrication to achieve controlled release.
The most forthright method for achieving taste-masking and controlled release with powders employs a two-step process in which a precursor particle is manufactured by a singular process, then coated with one or more layers containing modified-release excipients. Precursor particles can either be milled API crystals, API co-mixed with inert bases, or 100% inert cores without API. Particles can be manufactured by any method, which include vibratory methods, congealing/spinning disk atomization, prilling, hot-melt extrusion, spheronization, aqueous dispersions, blending/bulking, electrohydrodynamic spraying, or spray drying.24-31 Component selection for the particle relies on manufacturing capabilities, desired target product profile, and API process stability. Desired physical properties, such as surface features, density, friability, and hardness will also dictate which manufacturing scenarios are feasible for each product. If taste-masking, controlled-release, or stability-enabling properties are required, the particle typically advances to subsequent layering steps using fluidized beds, Würster coaters, spray/pan coating, or coacervation.20,32-35 Materials for the secondary coating steps are designated for reasons appropriate for precursor particles and patient use, such as material compatibility, controlled-release behavior, and stability. The final dosage form, typically granules in the 300-500-μm diameter range, can then be re-suspended, packaged in sprinkle packs or breakable capsules, placed in dissolving tongue strips, co-lyophilized with other materials for orally disintegrating tablets, or reconstituted in liquids.
The approach of manufacturing controlled-release powders by adding multiple coating steps to API-rich precursor particles is an accepted “way-of-life” for powder dosage forms. These techniques are, however, divergent from state-of-the art approaches that focus on chemical modification of the API and/or substrate using ion exchange resins.36-38 The main advantages that these methods can yield are liquid stability and deterring abuse of scheduled APIs, such as opiates and amphetamines. While innovative, drug complexation employs a number of manufacturing steps and quality control aspects that far surpass that of simple bead layering and may still require a final coating step.20,32-35 Thus, it is not surprising that pharmaceutical companies and CDMOs are investigating less complex chemistry and single-step manufacturing methods for producing controlled-release powders with pre-incorporated coatings.39-44
PRECISION PARTICLE FABRICATION® TECHNOLOGY: A NEXT-GENERATION CONTROLLED-RELEASE POWDER APPROACH
As demand grows for patient-friendly dosage forms, next-generation powder technologies offer the opportunity to provide not only operational efficiencies but speed to market by acting as a foundational building block for multiple format types. This building block approach reduces the development time associated with reformulating for multiple format types and therefore simplifies product lifecycle management for companies looking to optimize market opportunities.
Orbis’ Optimμm® platform leverages its Precision Particle Fabrication® technology to deliver oral pharmaceutical products that have both controlled-release and taste-masked attributes. Unique to other commonly used processes, Orbis’ controlled-release capabilities are achieved in a single manufacturing step and do not require additional coating steps to achieve taste-masking, extended release, or modified release. Figure 1 demonstrates how the Optimμm technology incorporates vibration with a scalable nozzle approach to create both microspheres and microcapsules in a single manufacturing step. The Optimμm technology produces uniform microspheres or microcapsules exhibiting a narrow size distribution offering precise control over particle morphology (e.g., porosity, coating thickness, etc.) and therefore precise control over release kinetics.39-44 These release kinetics can include extended- or modified-release options. The flexibility of the Optimμm technology allows for the production of a wide range of particle materials, sizes, and desired characteristics in a single-step process that scales linearly by simply adding additional nozzle heads. Scale-up has been successfully validated with the commissioning of Orbis’ cGMP-compliant suite (Figure 2).
Precisely controlled particle size enables key points of differentiation for those products leveraging the Optimμm technology. For example, the elimination of fines controls dose dumping and minimizes taste perception of the API improving palatability. Additionally, the consistency of the particles allows for more reliable and predictable dosage forms with batch-to-batch reproducibility for improved quality control. This consistency also creates a development workspace in which changes in attributes, such as particle size or API loading, create predictable dissolution outcomes reducing the number of iterations to reach a target product profile. This approach can be ideal for matching existing dissolution profiles for differentiated 505(b)(2) pathways. It also may serve in extending product life by limiting generic entrants as formulations leveraging Precision Particle Fabrication technology are difficult to replicate utilizing traditional technologies.
In addition to taste-masking and extended-release applications, Orbis’ novel core shell technology approach enables modified-release options, such as enteric or reverse-enteric delivery in a format-flexible powder. Figure 4 highlights in vitro results for an enteric release approach in which API release is limited to less than 10% for 2 hours in gastric conditions with immediate release when exposed to neutral pH similar to the small intestine. This modified-release approach is accomplished by fabricating a microcapsule with an engineered shell of uniform thickness designed for the target release specifications. Efficiencies are gained by eliminating secondary coating steps because Orbis’ fabrication process requires only one step.
Not only does Orbis’ technology offer a tightly controlled size distribution of its microspheres and microcapsules, it also offers a broad range of size options to address the needs of different formats. Typically, for oral applications, particle size ranges from 150 μm to 300 μm for optimal palatability. The Optimμm technology offers size ranges as low as 90 μm, eliminating the particle size limitations associated with some other powder technologies. As a melt-based process that is completely solvent-free, it also eliminates undesirable additives that can be associated with controlled-release technologies.
CONCLUSION
Providing formulations and treatments that improve clinical outcomes, for special populations, such as pediatric and geriatric patients, has been routinely noted as an area in need of improvement for pharmaceutical companies and providers.7-16,19-21,25,45-47 As the pharma landscape shifts from an environment of high-risk, high-reward blockbuster drugs to efficiency, economy, and ensuring patient outcomes, demand for technologies that can provide an efficient approach to product lifecycle management will increase. Controlled-release powders offer a flexible and efficient approach to addressing a multitude of patient populations, while also improving compliance. This “other solid dosage form,” offers the opportunity to unlock incremental value for those seeking differentiation in an increasingly competitive marketplace.
REFERENCES
1. Brown MT, Bussell JK. Medication Adherence: WHO Cares? Mayo Clinic Proceedings. 2011;86(4):304-314.
2. Mennella JA, et al. The bad taste of medicines: overview of basic research on bitter taste. Clin Therapeut. 2013;35(8):1225-1246.
3. Jayanthi B, Manna P. Per oral extended products – an overview. J App Pharm Sci. 2011;1:50-55.
4. Sansom L. Oral extended releas products. Aust Prescr. 1999;22:88-90.
5. PharmaCircle. 2016; Available from: www.pharmacircle.com.
6. Harb J. Why so many pills are TOO BIG to swallow: and why it’s safe to crush or cut up some, but not others. 2015. http://www.dailymail.co.uk/health/article-3151463/Why-pills-BIG-swallow-s-safe-crush-cut-not-others.html.
7. Bergstrom D, McNally E, Freeman S. The growing pediatrics market. Pharm Exec. 2004. http://www.pharmexec.com/growingpediatrics-market.
8. Bhardwaj S. Palatable Pharmaceutical Compositions. 1996. SmithKline Beecham Corporation.
9. Dickens D, Sinsabaugh D, Fahner J. Characteristics of pediatric chemotherapy medication errors in a national error reporting database. Cancer. 2008;112(2):445-6; author reply 446.
10. Engelen L, et al. Relating particles and texture perception. Physiol Behav. 2005;86(1-2):111-117.
11. Imai E, Hatae K, Shimada A. Oral perception of grittiness. J Textural Studies. 1995;26:561-576.
12. Matsui D. Current issues in pediatric medication adherence. Paediatr Drugs. 2007;9(5):283-288.
13. Milne C, Bruss J. The economics of pediatric formulation development for off-patent drugs. Clin Ther. 2008;30(11):2133-2145.
14. Rocca J, Park K. Oral drug delivery: prospects and challenges. Drug Development & Delivery, 2004;4(4):52-57.
15. Sugao H. Taste masking of bitter drug powder without loss of bioavailability by heat treatment of wax-coated microparticles. J Pharmaceut Sci. 1997;87(1):96-100.
16. Tyle P. Effect of size, shape and hardness of particles in suspension on oral texture and palatability. Acta Psychol (Amst). 1993;84(1):111-118.
17. Osterberg L, Blaschke T. Adherence to medication. NEJM. 2005;353(5):487-497.
18. Schier J, et al. Fatality from administration of labetalol and crushed extended-release nifedipine. Pharmacother. 2003;37(10):1420-1423.
19. Cram A, Bartlett J, Heimlich J. Oral multiparticulates as a flexible solid dosage form approach for paediatric use. BioPharm Asia. 2013.
20. Lopez F, et al. Formulation approaches to pediatric oral drug delivery: benefits and limitations of current platforms. Expert Opinion Drug Del. 2015;12(11):1727-1740.
21. Ivanovska V, et al. Pediatric drug formulations: a review of challenges and progress. Pediatrics. 2014;134(2):361-372.
22. Maalouf N. Developing Patient-Centric Drug Formulations to Meet Patient Needs. 2013. PLG Business Dev Licensing J 20 Available at: http://www.adarepharma.com/wp-content/uploads/2015/02/Nadines_PLG_Paper_2013.pdf (accessed April 2016).
23. Vummaneni V, Nagpal D. Taste masking technologies: an overview and recent updates. Int J Res Pharmaceut Biomed Sci. 2012;3(2):510-525.
24. Ambike A, Mahadik K, Paradkar A. Spraydried amorphous solid dispersions of simvastatin, a low Tg drug: in vitro and in vivo evaluations. Pharmaceut Res. 2005;22(6):990-998.
25. Cloupeau M, Prunet-Foch B. Electrohydrodynamic spraying functioning modes: a critical review. J Aerosol Sci. 1994;25(6):1021-1036.
26. Eldem T, Speiser P, Hincal A. Optimization of spray-dried and congealed lipid micropellets and characterization of their surface morphology by scanning electron microscopy. Pharmaceut Res. 1991;8(1):47-54.
27. Gharsallaoui A, et al. Applications of spraydrying in microencapsulation of food ingredients: an overview. Food Res Int. 2007;40(9):1107-1121.
28. Hancock B, et al. Pharmaceutical powders, blends, dry granulations, and immediate-release tablets. Pharmaceut Tech. 2003:64-80.
29. Passerini N, et al. Evaluation of melt granulation and ultrasonic spray congealing as techniques to enhance the dissolution of praziquantel. Int J Pharmaceut. 2006;318(1-2):92-102
30. Vehring R. Pharmaceutical particle engineering via spray drying. Pharmaceut Res. 2007;25(5):999-1022.
31. Yurteri C, Hartman R, Marijnissen J. Producing pharmaceutical particles via electrospraying with an emphasis on nano and nano structured particles – a review. KONA Powder and Particle Journal. 2010;28:91-115.
32. Dewettinck K, Huyghebaert A. Fluidized bed coating in food technology. Trends in Food Science & Technology. 1999;10(4-5):163-168.
33. Gouin S. Microencapsulation: industrial appraisal of existing technologies and trends. Trends in Food Science & Technology, 2004;15(7-8):330-347.
34. Jono K, et al. A review of particulate design for pharmaceutical powders and their production by spouted bed coating. Powder Technology. 2000;113(3):269-277.
35. Sastry S, Nyshadham J, Fix J. Recent technological advances in oral drug delivery – a review. Pharmaceutical Science & Technology Today. 2000;3(4):138-145.
36. Elder D. Pharmaceutical applications of ion exchange resins. J Chem Edu. 2005;82(4):575.
37. Fazal U, Khan S. Therapeutic applications of ion exchange resins. In: Ion Exchange Technology II: Applications. Inamuddin D, Luqman M, eds. Springer Netherlands: Dordrecht. 2012:149-168.
38. Pande S, Kshirsagar M, Chandewar A. Ion exchange resins: pharmaceutical applications and recent advancement. Int J Adv Pharm Sci. 2011. http://www.arjournals.org/index.php/ijaps/article/view/308.
39. Berkland C, et al. Monodisperse liquid-filled biodegradable microcapsules. Pharmaceut Res. 2007;24(5):1007-1013.
40. Pack D, et al. Three-month, zero-order piroxicam release from monodispersed double-walled microspheres of controlled shell thickness. J Biomed Mater Res Part A. 2004;70A(4):576-584.
41. Pack D, Berkland C, Kim K. Fabrication of PLG microspheres with precisely controlled and monodisperse size distributions. J Controlled Rel. 2001;73(1):59-74.
42. Pack D, Berkland C, Kim K. PLG microsphere size controls drug release rate through several competing factors. Pharmaceut Res. 2003;20(7):1055-1062.
43. Pack D, et al. Precise control of PLG microsphere size provides enhanced control of drug release rate. J Controlled Rel. 2002;82(1):137-147.
44. Pack D, et al. Microsphere size, precipitation kinetics and drug distribution control drug release from biodegradable polyanhydride microspheres. J Controlled Rel. 2004;94(1):129-141.
45. Best Pharmaceuticals for Children Act, in Public Law 107-1092002: Washington, DC.
46. Pediatric Research Equity Act, in Public Law 108-1552003: Washington, DC.
47. Regulation No. EC 1901/2006, E.P.a.t.C. EC, Editor 2006: Brussels.
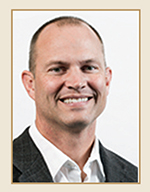
Dr. Cory Berkland is the Co-founder and CSO of Orbis Biosciences. He has been developing microencapsulation and drug delivery capabilities for more than a decade. He earned his PhD in Chemical and Biomolecular Engineering from the University of Illinois, Urbana-Champaign, where he co-invented and developed the Orbis technology. Dr. Berkland is also a Professor of Pharmaceutical Chemistry and Chemical and Petroleum Engineering at The University of Kansas.
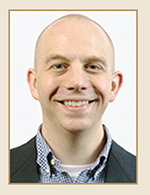
Dr. Nathan Dormer is Vice President of Research & Development at Orbis Biosciences. He has more than a decade of experience developing a variety of controlled-release solutions using microsphere techniques. He earned his BS in Chemical Engineering from The University of Kansas before completing his PhD in Bioengineering with Honors from The University of Kansas with NIH-sponsored training in drug delivery and protein stability. He has authored a number of publications and book chapters relating to microsphere encapsulation and has direct experience formulating dozens of active pharmaceutical ingredients.
Total Page Views: 6436