Issue:March 2014
CELL THERAPIES - The Challenges & Possible Solutions for Transferring Cell Therapy From the Bench to the Industry
INTRODUCTION
In the past decade, it has been proven that cell therapy works in the lab, in preclinical settings, and in small-scale clinical trials. The need for large quantities of cells with high quality becomes crucial as product candidates advance into clinical trials and early commercial products. Throughout recent years, many companies had invested efforts in developing culturing technologies that will allow large-scale culturing and manufacturing of cells. These technologies are evolving from tissue culture dishes and flasks to high-end, fully controlled bioreactor systems, which will allow production of large quantities of cells under cGMP (Current Good Manufacturing Procedures). The challenge becomes even bigger when looking at “off-the-shelf” allogeneic therapy. When mature, the industry will face an even larger challenge of downstream processing of the cell products. The following will discuss the significant emerging challenges in downstream processing of cell therapies focused mostly on allogeneic therapies.
LOT SIZE
Based on the publicly available information, the dose range for cell therapy clinical treatment can raise up to 600 million cells per treatment in repeated dosages. For this exercise, let’s assume a dose range of 300 million cells per patient and 100,000 patients a year. Taking in to consideration quality control and testing, the gross amount of cells that should be manufactured to meet such a need will be 50% higher. Therefore, 150,000 x 300 million cells = 45 trillion cells annually, resulting in a minimal batch size of 1 trillion cells. Once understanding the quantities needed to become an industry, monolayer, traditional culturing systems, such as 10 or 40 layers, become irrelevant as one will need more than 1000 trays of 40 layers cultured simultaneously. Using traditional and multi-layer culturing systems has even larger drawbacks that include very high cost of goods as well as very large infrastructure and labor costs. Regardless of the cost, the most problematic issue of such technology would be the quality of the cells. This quality would be hindered by the very long processing times, high variation associated with different culturing vessels, different incubators, different processing and stalling times, and mostly by the variation due to handling of the cells and vessels. Therefore, the only true scalable technology for culturing cell therapies for allogeneic therapies would be large bioreactors.
BIOREACTORS
Bioreactors are vessels that can grow cells in a controlled and monitored manner with a high volume-to-surface ratio, allowing culturing cells in high quantities per vessel. These technologies range from automated, closed system mono-layer surfaces, throw hollow-fiber culture surfaces, and micro-carrier based systems all the way to packed bed three-dimensional surfaces. As an example, the Fibra-cell packed bed technology used to culture cells for the protein industry has been adapted for cell therapy culturing and modified by Pluristem Therapeutics. This technology allows for the culturing of approximately 1 trillion cells in three to four 75-litter reactors. This technology leap makes the growing of large numbers of cells feasible and very cost effective. An additional advantage of reactors lay in the fact that they are monitored and tightly controlled, allowing optimal culture conditions, resulting in high yields and low variance between vessels and batches. Once the industry matures, it is safe to assume that the bioreactor technology will become the main stream culturing technology that allows for the large-scale manufacturing of cells.
CELL HARVESTING
The first challenge facing bioengineers today is cell harvesting from the reactors and carrier systems. Adherent cells attach to the carrier surface and can only be detached by enzymatic digestion of the adhesion proteins. Detaching the cells is usually not sufficient, and additional physical force has to be added. This force has to be strong enough to detach the cells but gentle enough not to hinder cell integrity or to break the carrier, resulting in carrier residues or dead cells. Overcoming the harvest yield challenge allows using reactor technologies for cell therapy. Several technologies utilize different physical force to overcome the challenge. For example, in the hollow-fiber technology (TerumoBCT), a controlled liquid cross flow process post enzymatic digestion will result in gentle lifting and harvesting of the cells. Alternatively, in the fiber-cell reactors (Pluristem Therapeutics), a custom made proprietary technology adds controlled agitation and movement to the enzymatic reaction, allowing high harvest efficiency with high cell viability. An additional approach for harvesting could be digesting the carrier itself. Many different technologies try to address this path by using biodegradable materials as carriers.
DOWNSTREAM PROCESSING
Downstream processing in cell therapy includes harvesting of cells out of the reactors and the carrier system, washing the cells, and eliminating all culture materials (such as media, serum, or ancillary ingredients), formulating, dosing, and cryopreserving the cells. Once the cells have been harvested, the clock begins to tick; cells are maintained outside their comforting environment without nutrition or suitable environmental conditions. The sensitivity of the cells to these stressful conditions differs, but the downstream processes are limited to a few hours. The downstream processes thus hold within them significant challenges with solutions that are becoming the cutting edge of cell therapy.
WASHING & CONCENTRATION OF CELLS
When the cells are in suspension, they should be washed out of the residual culture media, serum, or ancillary materials. The classical method of washing and concentrating cells is the use of batch centrifugation, which palate the cells to the bottom of a tube using G force (normally at about 1000 g). The media will then be discarded and the cells suspended using a pipet and addition of wash buffer. This process is repeated several times until reaching the expected clearance levels. Nevertheless, this process is very stressful to the cells, time consuming, and performed in tubes that have to be opened in order to replace the buffers and re-suspend the cells. These open manipulations amplify the risk of microorganism contamination to the final product, thereby adding risk to the patient. A few technologies exist that allows a closed system with continuous flow cell washing, some of which are based on blood separation technologies but are limited in their scalability. Alternatively, tangential-flow filtration (TFF) systems that have been used in the industry for protein concentration and diafiltration have been modified by several companies, such as Lonza, for use in cell therapy. However, its scalability limits have to be overcome, and in some cases, such technologies are stressful for the cells. An evolving processing approach has been developed by KBI Biopharma called the Ksep centrifuge. This technology uses two forces, working one against the other, the flow of the cells suspension or media and the centrifugation force. Cells are pumped continuously into a chamber that rotates at high speed. Through the balance of centrifugal and fluid flow forces, the kSep® retains the cells as a concentrated fluidized bed under a continuous flow of media or buffer. This allows washing and concentrating the cells with minimal stress using a closed and automated system. One additional and critical advantage is its scalability. To date, there is a commercially available model that can handle more than 1 billion cells per run.
CELLS SEPARATION & PURIFICATION
Cell separation is usually achieved by exploiting differences in basic cell properties, such as size, density, expression of molecular markers, or surface properties. The application of separation for large-scale production requires technologies capable of highly efficient cell purification using a completely closed environment in a short process time in order to maintain the cell’s quality. To meet these requirements, Milteny Biotec developed a closed and sterile system (CliniMACS®) based on magnetic-activated cell sorting, which allows for automated cell separation to a relatively large scale (up to 1.2 × 1011 initial cells). Biosafe developed the Sepax® technology based on a different separation principle termed Ficoll density gradient separation. The Sepax® is a GMP automated mononuclear cell isolation technology for umbilical cord blood or other sources. This kind of equipment can be used directly at the point of care (for autologous treatment) or during the isolation process of cell banking (for allogeneic treatment). Another approach for in-process cell separation is the use of aptamers. Aptamers are nucleic acid molecules generated by combinatorial chemistry and have the ability to bind specifically to molecular targets. Aptamers can be easily modified by attaching magnetic beads introduced to the cells using automated processes. Due to their low cost of production when compared with monoclonal antibodies, aptamers may contribute to a wider application of high -resolution affinity-based separation techniques in cell manufacturing, which would be very difficult with the currently available antibody technology. Different microfluidic separation devices based on filtration and sedimentation, affinity-based methods have also been developed for lab-scale production. However, in order to process large amounts of cells using these technologies, a scale-out method is needed that increases the risk of inconsistency between the devices.
FINAL FORMULATION & FILLING
The formulation of the cells prior to cryopreservation is conducted by determining the cell concentration and adding the final formulation additives to the suspension. This means that samples must come out of the suspension and material in it. Opening the container for the sampling and adding formulation additives increases the risk for contamination of the product at its final stage. As lot sizes increase, maintaining a homogenous cell’s suspension and relatively short process time will be difficult. Therefore, a vast amount of development has been invested in developing closed system sampling, adding and mixing the formulated cells. Most of the final formulation technologies are custom made per product. Final product dose and filling step duration is defined by lot size and the number of cells per dose. Currently, most of the cell therapy’s final product packagings are traditional blood bags. This type of packaging is suitable for small lot sizes in the range of several hundred doses per lot. Larger lots will require a shift to pharmaceutical vials and compatible filling automation in order to decrease process duration and maintaining cell quality. The use of new plastic vials from West or Aseptic Technologies coupled with traditional pharmaceutical fill line automation can enable the processing of lot sizes in the several hundred to several thousand doses per lot using the same scalable technology.
Cells that are kept cryopreserved are maintained in liquid nitrogen, which is 196°C and thawed rapidly in a 37°C water bath. Such a significant temperature change is very stressful to most materials, thus the containers are very limited to small infusion bags, plastic tubes with a screw-on cap, or the Aseptic technology patented vials. The disadvantage of bags is that their handling is not trivial. Once frozen, they are very sensitive and break easily. Furthermore, the cells freeze in a thin layer, making them very sensitive to temperature changes that might accrue during shipment or prior to the intended thawing. The plastic screw-on cap vials are commonly used in cell culturing and preservation, but they require opening the cap for filling and extracting the cells, which can lead to contamination of the cells and result in risk to the patient. Commonly used septum vials do not survive the liquid nitrogen freeze-thaw cycle as the different material comprising the vial and septum react differently to the temperature, resulting in leaks or brakes in the glass and contamination. Aseptic technologies had developed a unique technology in which a plastic septum vial is pre-closed together and can survive the cycle without leaking. In order to fill the vial, a needle is inserted through the septum, and the cells are pumped in. The septum is then sealed using a laser beam.
SHIPMENT HANDLING & DELIVERY
Because cells are cryopreserved, the shipment and handling is not straight forward. The cells must stay frozen, and the time they can remain outside of the nitrogen is very short. Temperature variations can significantly shorten their shelf-life. Therefore, shipment and storage must be done in a controlled manner with tight monitoring.
One of the most critical steps in the survival and viability of the cells is the thawing process. Generally, the thawing must be done rapidly in a water bath with gentle mixing to eliminate temperature gradients, resulting in water crystallization and cell damage. Water baths are an efficient way to thaw cells as water is a very good heat conductor. Nevertheless, water could be a risk factor for contamination, and there is a large variation in the way different people mix the cells during the thawing that results in variation of cell viability. Low cell viability will lead to low efficacy. Therefore, companies, such as Pluristem Therapeutics, have developed a custom made thawing device that can automatically thaw the cells in a controlled and uniform manner. Such a device promises high-quality cells delivered to the patient.
COST OF GOODS
Cell therapy is an expensive but promising therapy. The cost of the therapy is influenced by the cost of goods manufactured, overhead and infrastructure costs, and logistic costs of shipment and handling. Cells culture media, both serum-based and chemically defined, is very expensive. Efficient culturing conditions that would produce high yields of cells, have a major influence on the cost. Tightly controlled Bioreactors with a high surface-to-volume ratio, such as Pluristem’s Fibra-cell-based reactors, are the key for highly efficient manufacturing and a reduced cost of goods. An efficient testing and downstream processing mechanism can significantly affect the yields and viability of the product and directly affect the cost of the final product. Therefore, the issue of cost should be considered upfront from day one. Efficient large-scale manufacturing should be implemented very early on in the development life cycle of the cell therapy. Having said that, most early stage cell therapy companies choose not to invest in process development or the optimization of manufacturing due to high initial investments. Only a handful of cell therapy companies see this issue as critical and address the manufacturing, cost, and scalability issues early on. One such company is Pluristem Therapeutics, which develops and manufactures cell therapy products that originate from the placenta.
To view this issue and all back issues online, please visit www.drug-dev.com.
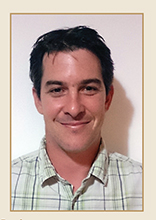
Lior Raviv is the Product Development Team Leader with the Process Development Department at Pluristem Therapeutics, where he develops new technologies for cells downstream process and final packaging. Prior to joining Pluristem, Lior was a R&D Analytical Researcher at Teva Pharmaceutical Industries Ltd. He earned his MMedSc in Pharmacology and his BSc in Biotechnology Engineering at the Ben Gurion University in Israel.
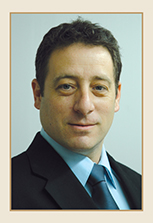
Dr. Ohad Karnieli earned his PhD in Biotechnology and Genetic Engineering from the Sackler School of Medicine at Tel Aviv University. Furthermore, Dr. Karnieli earned his MBA from the graduate school of Management at the Haifa University. In his PhD, Dr. Karnieli developed insulin-secreting cells from human mesenchymal stem cells using genetic modulations. Prior to joining Pluristem, Dr. Karnieli served as the General Manager of High Tech Lipids, an innovative IV nutrition company; the Vice President of Research and Development in an innovative nanobiotechnology start-up; and as the Vice President, Head of the Biomedical division at Goji solutions, where medical devices are developed using radiofrequency technology. Dr. Karnieli is the Founder of Karnieli Ltd., a leading molecular diagnostic and development lab.
Total Page Views: 3967