Issue:May 2015
BIOTHERAPEUTICS DEVELOPMENT - The Role of Flow Electroporation in Vaccine Development
INTRODUCTION
As the largest known outbreak of Ebola in human history has raced through West Africa killing thousands of people, big pharma and start-ups alike have searched for a vaccine to this deadly virus. Even if a vaccine were to be found in the near future, it could be months to years before it is commercially available because vaccine development and production is a lengthy and costly process. But, new technologies are available, and biotherapeutic development and production of vaccines can be accelerated.
Recognizing the need for rapid and reliable vaccine production, researchers have looked to recombinant technologies to develop innovative types of vaccines and new cell culture production methods that offer shorter lead times and greater production flexibility while maintaining vaccine safety.1-3 Newer engineered vaccine modalities range from therapeutic antibodies, subunit vaccines, virus-like particles (VLPs), and virus-like replicons particles (VRPs) to virus-mediated gene therapy. Each of these engineered vaccine types – whether a simple therapeutic protein or complex modification of patient cells – requires the introduction of recombinant nucleic acids into a cell line or ex vivo patient-isolated cells via transfection, viral transduction, and/or creation of stable cell lines. While the downstream processing is vaccine-dependent, a single unifying platform for upstream cell transfection could significantly reduce development timelines and production costs. For more than two decades, stable cell lines have been the standard for biotherapeutic protein production. However, their creation is a time-consuming, costly, labor-intensive process and is not feasible for all vaccine applications. As a result, researchers have turned to transient gene expression (TGE) as a means of more cost-effective protein production, particularly during early development and preclinical stages.4,5 While TGE offers a means of rapidly expressing proteins, not all transient expression methods generate the required amount of protein or fulfill the necessary requirements for broad use throughout vaccine development and production. One transient transfection technology (flow electroporation, a proprietary technology from MaxCyte, Inc.) not only meets all the conditions but can lead to significant resource and time savings.
Flow electroporation is a universal, regulatory-compliant transient transfection technology that provides a practical solution to the time, labor, and cost challenges of developing stable cell lines and baculovirus-based expression while overcoming the flexibility and scalability limitations associated with other transient transfection methods. Flow electroporation efficiently (co)transfects cells with DNA, RNA, siRNA, and cell lysates without requiring specialized constructs, engineered cells, media additives, or chemical reagents. It transfects from 5E5 cells in seconds up to 2E11 cells in less than 30 minutes.
With more than a dozen adherent- or suspension-adapted cell lines currently in use by vaccine manufacturers, there is a need for cell type flexibility.1,6 MaxCyte electroporation consistently results in high levels of transfection efficiency and cell viability for a wide range of cells, including CHO, MDCK, BHK-21, Vero, NS0, insect cells, and other cell lines commonly used for bioproduction. Moreover, cell immunotherapy using modified patient-isolated primary cells requires high transfection efficiency and low cell toxicity while meeting stringent sterility and safety needs.
As well as its cell type flexibility and scalability, flow electroporation is extremely easy to use with a preloaded library of optimized electroporation protocols. The combination of features adds up to a technology that is especially attractive for rapid response vaccine generation for pandemic, biodefense, or even seasonal influenza. From antibody production to lentivirus production, flow electroporation can accelerate vaccine development and production. To exemplify this, five case studies are presented below to show the role of flow electroporation in vaccine development.
GRAM-SCALE CHO ANTIBODY PRODUCTION
Therapeutic monoclonal antibodies (mAbs) are the predominant component of biotherapeutic development pipelines for a range of clinical indications, including inflammatory disorders, cancers, and infectious diseases. mAbs can be used as prophylactic or therapeutic interventions via a variety of means ranging from targeted radioimmunotherapy to anti-idiotype immunization.
The amount of protein required throughout mAb development varies from low milligram to multi-gram quantities. Despite advances in transfection methods and culture optimization, the majority of CHO cell-based TGE methods have reported antibody titers ranging from 2 to 250 mg/L upon full optimization.7-11 More recently, there have been reports of 2 g/L after extensive work engineering a CHO cell line and optimizing culture conditions, something that is not feasible when a rapid response is needed.
Large-scale flow electroporation studies have demonstrated the ability to rapidly produce secreted antibody titers >400 mg/L, which can exceed >1 g/L with optimization of post transfection culture conditions, such as cell density, feeding conditions, media additives, and culture temperature.12 This translates into the production of multi-gram quantities of antibodies within days of transfection. With a moderate amount of post transfection process development, flow electroporation has resulted in antibody titers greater than 1.2 g/L, producing greater than 3 g of antibody from a non-engineered CHO culture of less than 3 L in 12 days (Figure 1). Further scale-up to biomanufacturing using the MaxCyte VLX® Large Scale Transfection System allows for transfection of up to 2E11 cells, which would equate to the production of more than 50 g of antibody within 2 weeks of a single transient transfection. The speed and simplicity of the process allows for rapid generation of quantities of antibody to meet rapid response needs.
HIGH-LEVEL SUBUNIT VACCINE EXPRESSION
Similar to inactivated virus vaccines, subunit vaccines are generally easy to administer, do not contain infectious material, nor require a cold chain for distribution. Unlike inactivated viruses, subunit vaccines contain only the antigen(s) that best stimulate a protective immune response. Subunit vaccine antigen(s) can be isolated directly from the pathogen, as is the case with the Haemophilus influenzae type b vaccine (parenteral form), or expressed recombinantly, like the current hepatitis B vaccine composed of the hepatitis B virus surface antigen (HBsAg). One advantage of recombinant expression is that the antigen(s) can be manipulated to increase immunogenicity, to elicit a more protective immune response, or to be expressed as peptides or fusion proteins.
Tests between flow electroporation and conventional methods have shown electroporation to be a rapid means of expressing recombinant antigens with superior performance. In CHO-S cells that were transfected with an HIV-1 gp145 expression plasmid via electroporation and PEI, the viability of PEI-transfected cells dropped off substantially by day 3, while >95% viability is maintained for 10 days after electroporation. Higher cell viability was reflected as 25-fold higher expression of the HIV-1 gp145 envelope protein based on volumetric titers.
RAPID RESPONSE VACCINES USING INSECT CELL VLP PRODUCTION
VLPs are a promising avenue of vaccination with an inherently higher level of safety compared to inactivated or live attenuated viral vaccines. These particles consist of one or more recombinantly expressed viral structural proteins that self-assemble into complexes that closely mimic the three-dimensional structure of native virus but lack the viral genome. VLPs have been produced for a variety of viruses, including influenza, and are an FDA-approved vaccine against human papillomavirus.3,13,14
VLPs are manufactured using an assortment of cells, including mammalian and insect cells. Insect cells are used because they are easy to culture with simplified cell growth that is readily adapted to high-density suspension and they post-translationally modify proteins in a manner similar to that of mammalian cells. While both transient transfection and recombinant baculovirus platforms are commonly used methods for insect cell protein expression, there are advantages to using electroporation.15,16
Baculovirus-mediated protein production is an extended, multi-stage process, despite development of specialized media and baculovirus vectors aimed at simplifying gene cloning and virus stock production. This 6- to 8-week process requires construction of expression plasmid(s), transfection of insect cells, viral stock preparation, and subsequent infection of insect cells, all prior to final production and purification of the recombinant protein(s) of interest.
In comparison to baculovirus, the MaxCyte STX® Scalable Transfection System, which directly transfects Sf9, Sf21, and SL3 cells with >90% cell viability and transfection efficiency levels, allows for rapid, high-level protein production within 3 days. In one experiment, Sf9 cells were transfected via electroporation with an expression construct encoding three VLP antigens that resulted in significant secretion of the VLP within 48 hours post transfection. In tandem, a baculovirus expression system was used to produce VLPs containing the identical three antigens. Analysis of VLPs following sedimentation through a sucrose cushion shows the presence of the three VLP antigens in all electroporation and baculovirus samples; however, baculovirus protein contaminants were also present in preparations of VLPs from baculovirus-infected cultures. This is consistent with the literature, which documents the propensity for baculovirus protein contamination, creating purification challenges, and yield loss.17,18 VLP production is streamlined with flow electroporation because there is no need to create a viral stock, go through several rounds of virus amplification, or remove contaminating baculovirus proteins during purification.
INCREASED EFFICIENCY OF ALPHAVIRUS VRP PRODUCTION
Alphaviruses have a broad cellular tropism, but preferentially infect dendritic cells, thereby acting as a delivery vehicle to professional antigen-presenting cells (APCs), which in turn stimulate both humoral and cellular immune responses. Alphavirus-derived particles, called virus-like replicon particles, represent a viable next-generation vaccine option, particularly against NIH Risk Group 3 viruses (such as H5N1, HIV, Ebola, etc) as low biocontainment facilities can be used to produce the non-pathogenic VRPs.
VRPs are produced by (co)transfecting cells with an alphavirus replicon RNA and helper RNAs or DNA plasmids expressing the alphavirus structural proteins, followed by harvesting of VRP-containing culture media. Many different cell lines are permissive to alphavirus infection, allowing a variety of cells to be used for VRP production. Vero and BHK are most common, but others including CHO and HEK have been used. Several VRPs produced via electroporation of Vero cells have entered into clinical studies.17
Alphavirus VRP experiments have been conducted in which the current “best practice” of transfection was compared to electroporation. Production of two VRPs was assessed: one expressing GFP and the second expressing the HIV gag protein. Electroporation led to 9X higher relative titers of both the GFP and HIV gag-expressing VRPs over the current best practice method.
STREAMLINED SCALE-UP OF LENTIVIRUS PRODUCTION
Lentiviruses, a subclass of retroviruses, are popular in vivo gene delivery vectors due to their unique ability to integrate into the host genome of non-dividing cells, the minimal immune response they induce, their reduced risk of insertional mutagenesis, and the long-term nature of their expression. Lentiviral vectors are developed by removing all the viral genes except those required in cis in order to complete a single round of replication. All other viral components are provided in trans during lentivirus stock production, and for biosafety considerations, the components are generally encoded by three to five separate plasmids
Due to the toxicity of vector components to the host cell, stable lentivector production cell lines are difficult to generate. Thus, lentivirus stocks are historically produced by transiently co-transfecting a host cell line with the multiple plasmids encoding the various lentivector components.18 Inefficiencies associated with chemical-based transfection methods, particularly when they are used for multi-plasmid co-transfection, typically restrict lentivector production to adherent cells, which are easier to transfect but are challenging to culture at large scale. Flow electroporation offers a rapid, fully scalable and cGMP-compliant alternative to chemical-based transfection methods that efficiently co-transfects suspension-adapted cells.
Flow electroporation has proven reliable performance. In large-scale production studies using a 4-plasmid lentiviral system, titers of approximately 38 ± 3.21 transducing units of virus per cell were achieved in three pilot qualification lots manufactured at a cGMP facility.19 These studies demonstrated the consistent, highly reproducible nature of flow electroporation.
SUMMARY
Two factors should be foremost when facing a rapid response situation: the need to accelerate development of vaccines and the need for a large quantity of protein. Flow electroporation can provide large quantities of protein using commercially available cell lines and standard processes. Furthermore, flow electroporation can accelerate development of antibodies, recombinant antigens, VLPs, VRPs, viral vectors, and cell immunotherapies, thereby reducing cost and shortening timelines. With unmatched quality, flexibility, and scalability, flow electroporation is a universal, cost-effective platform that supports the full range of biotherapeutic and vaccine development activities.
To view this issue and all back issues online, please visit www.drug-dev.com.
REFERENCES
1. Whitford W. Using Disposables in Cell-Culture-based Vaccine Production. Bioprocess Int. 2010;8(S4):S20-S27.
2. Reisinger KS, Block SL, Izu A, et al. Subunit Influenza Vaccines Produced from Cell Culture or in Embryonated Chicken Eggs: Comparison of Safety, Reactogenicity, and Immunogenicity. J Infect Dis. 2009;200(6):849-857.
3. Lambert L, Fauci, A. Influenza Vaccines for the Future. N Engl J Med. 2010;363(21):2036-2044.
4. Agrawal V, Bal M. Strategies for Rapid Production of Therapeutic Proteins in Mammalian Cells. Bioprocess Int. 2012;10(4):32-48.
5. Baldi L, Hacker DL, Adam M, Wurm FM. Recombinant Protein Production by Large-scale Transient Gene Expression in Mammalian Cells: State of the Art and Future Perspectives. Biotechnol Lett. 2007;29(5):677-684.
6. Whitford W, Fairbank A. Considerations in Scale-up of Viral Vaccine Production. BioProcess Int. 2011;9(S8):S16-28.
7. Bollin F, Dechavanne V, Chevalet L. Design of Experiment in CHO and HEK Transient Transfection Condition Optimization. Prot Expr Purif. 2011;78(1):61-68.
8. Galbraith DJ, Tait A, Racher AJ, et al. Control of Culture Environment for Improved Polyethylenimine-Mediated Transient Production of Recombinant Monoclonal Antibodies by CHO Cells. Biotechnol Progr. 2006;22(3):753-762.
9. Liu C, Dalby B, Chen W, et al. Transient Transfection Factors for High-Level Recombinant Protein Production in Suspension Cultured Mammalian Cells. Mol Biotechnol. 2008;39(2):141-153.
10. Ye J, Kober V, Tellers M, et al. High-level Protein Expression in Scalable CHO Transient Transfection. Biotechnol Bioeng. 2009;103(3):542–551.
11. Rajendra Y, Kiseljak D, Baldi L, et al. A Simple High-yielding Process for Transient Gene Expression in CHO cells. J Biotechnol. 2011;153(1-2):22-26.
12. Steger K, Brady J, Wang W, et al. CHO-S Antibody Titers >1 Gram/Liter Using Flow Electroporation-Mediated Transient Gene Expression Followed By Rapid Migration to High-Yield Stable Cell Lines. J Biomol Screen. Accepted for publication.
13. Landry N, Ward BJ, Trépanier S, et al. Preclinical and Clinical Development of Plant-Made Virus-Like Particle Vaccine against Avian H5N1 Influenza. PLoS ONE. 2010;5(12):e15559.
14. US Food and Drug Administration. Complete List of Vaccines Licensed for Immunization and Distribution in the US. 2014. http://www.fda.gov/biologicsbloodvaccines/vaccines/approvedproducts/ucm0 93833.htm. Updated September 29, 2014. Accessed May 1, 2014.
15. Liu F, Wu X, Li L, et al. Use of Baculovirus Expression System for Generation of Virus-like Particles: Successes and Challenges. Prot Expr Purif. 2013;90(2):104-116.
16. Kushnir N, Streatfield SJ, Yusibov V. Virus-like Particles as a Highly Efficient Vaccine Platform: Diversity of Targets and Production Systems and Advances in Clinical Development. Vaccine. 2012;31(1):58-83.
17. Vander Veen RL, Harris DJH, Kamrud KI. Alphavirus Replicon Vaccines. Anim Health Res Rev. 2012;13(1):1-9.
18. Howarth JL, Lee YB, Uney JB. Using Viral Vectors as Gene Transfer Tools. Cell Biol Toxicol. 2010;26(1):1-10.
19. Witting SR, Li L-H, Jasti A, et al. Efficient Large Volume Lentiviral Vector Production Using Flow Electroporation. Hum Gene Ther. 2012;23:243-249.
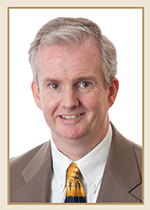
Dr. James Brady is Director of Technical Applications at MaxCyte, Inc, where he has worked since 2004. Dr. Brady earned his BS in Biology from the College of William and Mary and his PhD in Genetics from Indiana University. He also completed post-doctoral training at the National Eye Institute of the National Institutes of Health.
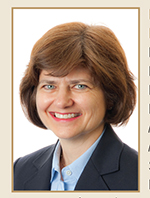
Dr. Karen Donato is Executive Vice President, Global Business Development and Marketing at MaxCyte, Inc. She earned her PhD in Chemical Engineering from Ohio University with broad experience in new business development, R&D, and operations in multinational, midsize, and start-up companies.
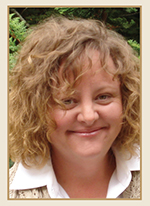
Dr. Krista Steger is President and Founder of Forge 4ward and a consultant to MaxCyte, Inc. She has more than 15 years of experience in global scientific marketing at leading biotechnology companies and start-ups. Dr. Steger earned her PhD in Cell and Molecular Biology at the University of Wisconsin-Madison.
Total Page Views: 3706