Issue:April 2017
ANTIMICROBIAL LIPIDS - Attenuating the Use of Medically Important Antimicrobial Drugs in Food-Producing Animals: What Role Can cGMP Lipids Play?
ABSTRACT
The US Food and Drug Administration (FDA) recently issued guidance intended to curtail the use of medically important antibiotics in agricultural applications. In the wake of these recommendations, the veterinary medicine community has mobilized to define and commercialize effective alternative pathogen controls. In this article, we draw attention to the scope of the challenge by highlighting some of the most significant, pathogen-borne diseases relevant to food-producing animals. We also review the antimicrobial properties intrinsic to midchain triglyceride lipolysis products, and present the question: What is the untapped potential of these safe-for-consumption, environmentally benign, and mechanistically privileged antimicrobial natural products
INTRODUCTION
“A definitive link (exists) between the routine, nontherapeutic use of antibiotics in food animal production and the crisis of antibiotic resistance in humans.”1 In 2012, as part of a campaign to reduce the selection pressure that drives the advancement of antibiotic-resistant bacteria strains, the FDA issued Guidance for Industry (GFI) #209, which aims to limit the agricultural use of antibiotics that have important human therapeutic applications,2 and defines two principles for judicious drug use in food-producing animals. Administration should be (1) limited to those uses that are considered necessary for ensuring animal health, and (2) limited to those uses that include veterinary oversight or consultation. As a corollary, in 2013, the FDA published means for voluntarily phasing out growth promotion indications for medically important antibiotics in accordance with GFI #209.3 In line with these recommendations, the veterinary health community is striving to bring alternative approaches for mitigating pathogen-borne diseases to bear while maintaining the industry’s ability to meet the nutritional demands of a growing global community.
Of course, finding alternative means for preventing, treating, or controlling pathogen-borne diseases in food-producing animals is no easy feat. Pathogenicity ties to a wide array of bacteria (e.g., Gram-negative, Gram-positive, both), can manifest in myriad disorders, and can impact every food-producing animal species. The permutations are daunting and, as a result, comprehensive solutions will continue to be difficult, if not impossible, to identify. The challenge is exacerbated by the fact that antibiotic drug innovation has significantly lagged behind the pace of resistance development.4,5 The following underscores some of the most pressing indications relevant to different agricultural livestock. Midchain triglycerides, in view of the antimicrobial properties of their lipolysis products, are also discussed as a potentially untapped resource for protecting the food supply and reducing the selection pressure that breeds microbe antibiotic resistance.
UNDERSTANDING THE BREADTH OF THE CHALLENGE
Perhaps the best way to communicate the scope of the selection pressure reduction/animal health protection conundrum is to call attention to some of the challenges recently presented in the open innovation forum. Stakeholders from the animal pharmaceutical industry (e.g., Elanco) have recently issued requests for proposals relevant to swine, poultry, and cattle health. The following case studies are by no means comprehensive, but they do serve to illustrate the breadth of the challenge.
Case Study 1: Ruminant Mastitis
Ruminant mastitis is an inflammatory reaction of udder tissue that is often attributable to bacterial infection. It is the most prevalent disease in dairy cattle in the United States, and is the costliest affliction for the dairy industry. Mastitis accounts for losses of approximately $200 per year, per cow.6 If the underlying infection persists unchecked, it can be fatal for the animal. Single pathogens or combinations thereof can spur mastitis. The Gram-positive bacteria staphylococci and streptococci are the most commonly observed causative pathogens, but Gram-negative bacteria, such as E. coli, have also been observed to cause the disease. Mycoplasma bovis is yet another pathogen of increasing relevance as a cause of mastitis. Absent the ability to broadly administer antibiotic mitigations for mastitis going forward – and in view of the 20% infection rate (despite antibiotic regime implementation) – alternative approaches are urgently needed for preventing, treating, or controlling mastitis in dairy cattle and other ruminants.7,8
Case Study 2: Porcine Ileitis
Proliferative enteropathy (PE, also known as ileitis) is one of the most prevalent and economically detrimental diseases affecting the global swine industry.9 Ileitis is caused by the bacterial pathogen, Lawsonia intracellularis. The Gram-negative bacterium infects the enteral epithelial cells of the animal, triggering hyperplasia and inflammation in the small and large intestines. To illustrate its economic impact, a recent study of a 735 sample population found an infection rate of 16.87%,10 and the cost to treat PE is estimated at $2 to $3 per pig ($15 in severe cases).11 Vaccination, antibiotic treatments, or combinations thereof are effective in the mitigation and treatment of natural and clinically mediated outbreaks of ileitis in pigs.10 GFI #209 and GFI #213 may limit, however, the continued use of streptogramin (eg, Virginiamycin), macrolide (eg, Tylosin), tetracycline (eg, chlortetracycline) and fluoroquinoline (eg, Enrofloxacin) class treatment options.11,12 Animal health stakeholders are therefore seeking to bolster the available solution set, which presently includes Enterisol® Ileitis vaccine and veterinary use-approved pleuromutilin class antibiotics (eg, Tiamulin).13,14
Case Study 3: Necrotic Enteritis in Poultry
Gram-positive Clostridium perfringens have been cited as one of the most common causes of food poisoning in humans, often resulting from insufficient poultry and meat cooking times and temperatures.15 In living food-producing fowl, necrotic enteritis is one of the most prominent manifestations of C. perfringens’ enterotoxins. The food safety implications, higher rates of mortality, and stunted animal growth associated with necrotic enteritis are estimated to cost the global poultry industry more than $2 billion annually.16,17 Until recently, food- and water-borne dispensation of antibiotics was adequate in keeping C. perfringens’ pathogenicity at bay. Because some of the administered classes of antibiotics saw shared use in human therapeutics, and because some were used principally for growth promotion, this preventive measure was discontinued. To meet the aspirations of GFI #209 and GFI #213 and protect the health of this integral food-producing animal category, new options are required to control the disease.
ANTIMICROBIAL LIPIDS’ RELEVANCE IN THE “POST-ANTIBIOTIC ERA”
The in vitro antimicrobial and antiviral potential of fatty acids and monoglycerides has been extensively documented in the primary and secondary scientific literature,18-20 with the phenomenon first having been documented (in the modern scientific era) in the late 19th century.21,22 Studies of the biological ramifications of ingesting triglycerides comprising these fatty acids and monoglycerides, however, are perhaps lesser known. For example, a series of inquiries correlating certain dietary lipids and a reduced incidence of pathogen-borne infectious diseases was the subject of unrelated research, which nevertheless seeds a potential approach to mitigating antibiotic resistance.
STUDIES REVEALING LIPIDS’ ANTIMICROBIAL PROPERTIES SPUR IMPORTANT QUESTIONS
The antibacterial contribution of fatty acid triglycerides in human colostrum and milk, and the mechanism by which these lipids realize their antibiotic potential, was defined as part of a broader initiative to rationalize higher infection resistance observed in breast-fed, versus bottle-fed, infants.23-26 In their seminal 1978 publication, Welsh and May observed that the lipolysis products of colostral lipids – in particular, monoglyceride and fatty acid fractions – were implicit resistance factors in human milk.27-29 Isaacs and coworkers further substantiated this finding, defining the role of endogenous lipases (lingual and gastric) in unmasking milk triglycerides’ antimicrobial activity in the gastric environment.30-32 Important contemporary research conducted by Kabara et al significantly expanded the understanding of fatty acid/monoglyceride structure-antimicrobial function relationships;33-37 the superior antibacterial potency of midchain acids and MAGs became clear. In view of this work, Isaacs et al went on to compare the lipase-mediated antiviral potential of infant formulas containing midchain triglycerides against those exclusively containing long chain triglycerides. MCT-containing formulas exhibited much more potent antimicrobial activity than those containing conventional dietary lipids.38-40 These studies raise important questions as to whether masked antimicrobial triglycerides (ie, midchain and particular structured triglycerides) can meaningfully reduce the incidence of pathogen-borne enteric diseases in agricultural animals.41
Is there an analogy to be established with observations of reduced infection occurrence in human infants? If the in vivo mechanism of coaxing certain lipid triglycerides’ antimicrobial activity holds, the answer is likely “Yes.”
ANTIMICROBIAL LIPIDS DEMONSTRATE THE SCOPE TO ADDRESS GRAM-POSITIVE & GRAM-NEGATIVE PATHOGENS
For a dietary or other adjunctive therapeutic strategy to have true utility preventing, treating, or controlling infections in animals, the antimicrobial lipids revealed during lipolysis would need to demonstrate broad antimicrobial efficacy. Indeed, Gram-positive and Gram-negative bacteria have both been shown to be susceptible to the membrane disruptive characteristics of monoglycerides and free fatty acids. Incidentally, midchain variants are the most frequently cited antimicrobial agents. Table 1 highlights specific species of bacteria and the fatty acid and/or monoglycerides that have been observed to inhibit their growth, in vitro. Studies conducted by Kabara,33-37 Bergsson,42 and McGuire43 demonstrate that Gram-positive staphylococci and streptococci, the most commonly implicated pathogens in bovine mastitis, succumb to the action of monoglycerides and fatty acids. Petra,44 Thormar,49 and Bergsson50 have collectively shown that Gram-negative bacteria including E. coli, H. pylori, various Campylobacter species, and P. aeruginosa are vulnerable to monocaprin. These examples of Gram-negative bactericidal activity bode well for enterally released MAGs’ and FFAs’ potential to control pathogens responsible for ileitis and necrotic enteritis. As can be gleaned from Table 1, triglyceride lipolysis products show a much broader range of antimicrobial activity than is presently discussed.
NASCENT ANTIMICROBIAL MCT & STRUCTURED LIPIDS ARE PRACTICAL IN USE
Examining the therapeutic effectiveness of masked antimicrobial lipids represents, perhaps, a simpler task than taking on other antibiotic attenuation strategies. Certainly, the scope pales in comparison to that of developing new classes of antibiotics for animal health applications and bringing them to market. To this point, MCTs (eg, C7-C13 fatty acid triglycerides) and structured triglycerides (eg, glyceryl tricaprylate/caprate/linoleate) have a well-established record of safe use in human and animal nutrition applications (Figure 1). Additionally, there is global precedence for the use of lipid-based excipients for enhanced drug delivery in human and veterinary medicine. Implementation approaches for liquid, semi-solid, and solid lipids have also been established, lending credence to the feasibility of this unique application. Lipids can be administered in solid feed formulations, in liquid feed formulations (eg, as dilute emulsions for enteral use), and in formulations designed for modified, targeted and/or controlled release. In the latter case, liposomal, solid lipid nanoparticle and parenteral systems would come in to play. Antimicrobial lipid delivery mechanisms, and the scope of antimicrobial activity of monoglycerides and fatty acids themselves, have been very well reviewed in recent years.18-20
AGRICULTURAL APPLICATIONS OF ANTIMICROBIAL LIPIDS ARE ALREADY UNDERWAY
Understanding the antimicrobial potential of monoglycerides and fatty acids – and triglycerides in conjunction with lipolytic enzymes – has, of course, spurred real-world application pursuits. For example, Hilmarsson et al evaluated the effect of glycerol monocaprate on broiler chickens that were deliberately, and naturally, exposed to the Gram-negative bacterium C. jejuni.51 The viable bacteria count in artificially contaminated water (initially, 6 log10 cfu) was observed to be reduced to undetectable levels upon treatment with glycerol monocaprate emulsions (0.6% monocaprin). No infection was observed in chickens provided treated water, and healthy growth was observed in the experimental group. In a nod to the utility of MCTs as masked antimicrobials, Aurousseau et al in 1984 reported an observed 40% growth rate increase in preruminant calves that were fed a glyceryl tricaproate- or tricaprylate-containing milk substitute formulation, as compared with a formula containing tallow lipids alone.52 The antimicrobial activity of the C6/C8 fatty acids released by gastric lipases in the gut rumen was cited as the principal rationale for the favorable growth outcomes. Perhaps most importantly, Diereck and Decuypere in 2002 methodically demonstrated the use of structured and midchain triglycerides in tandem with lipolytic enzymes to favorably impact the gut flora of piglets.53,54 These studies reinforce the notion that enterally released fatty acids and monoglycerides constitute a legitimate and effective approach to controlling myriad enteric infections in food-producing animals.
ANTIMICROBIAL LIPIDS’ ALIGNMENT WITH THE PRINCIPAL OF DOING NO HARM
Safe for Animal & Human Consumption
As previously mentioned, midchain and structured triglycerides have a well-established record of safe use in food, nutrition, and pharmaceutical applications – human and animal. It should also be underscored that antimicrobial monoglycerides and fatty acids (ie, triglyceride lipolysis products) are Generally Recognized as Safe (GRAS) by the FDA per CFR § 184.1505 and CFR § 172.860, respectively.
Environmentally Friendly
Categorically, midchain and structured triglycerides demonstrate ready biodegradability. This is a function of the fact that they comprise hydrolytically unstable ester bonds, and are composed of natural product fatty acids commonly encountered in the environment and in a wide variety of biological systems.
No Exertion of Selection Pressure
The membrane disruption mechanism underlying FFA and MAG antimicrobial properties42,50,55,56 does not contribute to the prevalence of antibiotic-resistant bacteria. These species are not understood to invoke the reproductive or metabolic interference mechanisms common to conventional antibiotic classes.
Scalability, Relevance
Midchain and structured triglycerides can be manufactured in a cGMP environment, on scales suitable for the agricultural animal health applications proposed herein.
SUMMARY
Organizations across the food-producing animal industry are proactively doing their part to address the specter of antibiotic resistance. Among them, the animal pharmaceutical industry continues to grapple with how they will continue to enable farmers’ delivery against the nutritional demands of a growing global community, while also winding down the use of antibiotics of mutual importance in human therapeutics. In this article, we have raised examples of focal point diseases that are driving the search for viable pathogen control alternatives, and have raised the important role that midchain and structured triglycerides can play in the effort.
REFERENCES
1. Clifford, J.; Khan, A.; Sharfstein, J. Hearing before the House Committee on Energy and Commerce, Subcommittee on Health. July 14, 2010. Antibiotic resistance and the use of antibiotics in animal agriculture. The authors represented the U.S. Food & Drug Administration, the U.S. Department of Agriculture, and the Centers for Disease Control and Prevention.
2. U.S. Food & Drug Administration. Guidance for Industry 213. December 2013. New Animal Drugs and New Animal Drug Combination Products Administered in or on Medicated Feed or Drinking Water of Food-Producing Animals: Recommendations for Drug Sponsors for Voluntarily Aligning Product Use Conditions with GFI 209. http://www.fda.gov/downloads/AnimalVeterinary/GuidanceComplianceEnforcement/GuidanceforIndustry/UCM299624.pdf. (RetrievedDec. 12, 2016).
3. U.S. Food & Drug Administration. Guidance forIndustry 209. April, 2012. The Judicious Use of Medically Important Antimicrobial Drugs in Food-Producing Animals. http://www.fda.gov/downloads/AnimalVeterinary/GuidanceComplianceEnforcement/GuidanceforIndustry/UCM216936.pdf. (Retrieved Dec. 12, 2016).
4. Cooper, M.A.; Shlaes, D. Nature 2011, 472, 32. Fix the antibiotics pipeline.
5. Shlaes, D.M. et al. Antimicrob. Agents Chemother. 2013, 57, 4605. The FDA reboot of antibiotic development.
6. Department of Animal Science. MacDonald Campus of McGill University. “Mastitis in Dairy Cows” 2003. https://web.archive.org/web/20030708113702/http://animsci.agrenv.mcgill.ca/courses/450/topics/13.pdf. (Retrieved December 27,2016).
7. Alternatives to antibiotics: Prevention or treatment of food-producing animals. InnoCentive Challenge ID: 9933897. Waltham, MA: InnoCentive, Inc. Posted December 20, 2016. For an additional example of important pathogen-precipitated cattle diseases, see reference 8.
8. Elanco, Inc. Liver Abscess formation in cattle. InnoCentive Challenge ID: 9933889. Waltham, MA: InnoCentive, Inc. Posted November 17, 2016.
9. Kroll, J.J. et al. Animal Health Res. Rev. 2005, 6, 173. Proliferative enteropathy: a global enteric disease of pigs caused by Lawsonia Intracellularis.
10. Alberton, E.L. et al. Acta Sci. Vet. 2011, 39, 943. Prevalence of porcine proliferative enteropathy associated to Lawsonia intracellularis in pigs slaughtered in Mato Grosso State, Brazil.
11. Boehringer Ingelheim Animal Health GmbH. Ileitis Technical Manual 3.0 2006, Ch. 6.2. Which antibiotics are most effective against ileitis? http://www.thepigsite.com/publications/2/ileitis/93/62-which-antibiotics-are-mosteffective-against-ileitis/. (Retrieved Dec. 13,2016).
12. Muirhead, M.R.; Alexander, T.J.L. Managing Pig Health: A Reference for the Farm, 2nd Ed. Carr, J. (ed.) 5M Publishing: United Kingdom, 2013.
13. Elanco, Inc. Prevention and Control of Enteropathy/Ileitis in Swine. InnoCentive Challenge ID:9933892. Waltham, MA: InnoCentive, Inc. Posted December 1, 2016. For an additional example of an important pathogen-precipitated cattle disease, see reference 14.
14. Elanco, Inc. Prevention and control of Streptococcus suis infections in swine. InnoCentive Challenge ID: 9933893. Waltham, MA: InnoCentive, Inc. Posted September 8, 2016.
15. Warrell et al. Oxford Textbook of Medicine, 4th ed.; Oxford, UK: Oxford University Press; 2003.
16. Elanco, Inc. Prevention, treatment or control of necrotic enteritis in poultry. InnoCentive Challenge ID: 9933891. Waltham, MA: InnoCentive, Inc. Posted October 14, 2016.
17. Elanco, Inc. Coccidiosis prevention and control in poultry. InnoCentive Challenge ID: 9933890. Waltham, MA: InnoCentive, Inc. Posted December 15, 2016.
18. Thormar, H.; Hilmarsson, H. Chem. Phys. Lipids 2007, 150, 1. The role of microbicidal lipids in host-defense against pathogens and their potential use as therapeutic agents.
19. Jackman, J.A. et al. Molecules 2016, 21, 305. Nanotechnology formulations for antibacterial free fatty acids and monoglycerides.
20. Dayrit, F.M. J. Am. Oil Chem. Soc. 2015, 92, 1. The properties of lauric acid and their signifi- cance in coconut oil. Clark. J.R. Botan. Gaz. 1899, 28, 289.
21. Thormar, H. Antibacterial Effects of Lipids: Historical Review (1881 to 1960), in Lipids and Essential Oils as Antimicrobial Agents; Hoboken, NJ: John Wiley & Sons, Ltd; 2011.
22. Cunningham, A.S. J. Pediatr. 1977, 90, 726. Morbidity in breast-fed and artificially fed infants.
23. Downham, M.A.P.S. et al. Br. Med. J. 1976, 2, 274. Breast-feeding protects against respiratory syncytial virus infections.
24. Winberg, J.; Wessmer, G. Lancet 1971, 1, 1091. Does breast milk protect against septicaemia in the newborn?
25. Adebonojo, F.O. Clin. Pediatr. 1972, 11, 25. Artificial vs. breast feeding: relation to infant health in a middle class American community.
26. Welsh, J.K.; Skurrie, I.J.; May, J.T. Infect. Immun. 1978, 19, 395. Use of Semliki Forest Virus to identify lipid-mediated antiviral activity and antialphavirus Immunoglobulin A in human milk.
27. Welsh, J.K.; May, J.T. J. Pediatr. 1979, 94, 1. Anti-infective properties of breast milk.
28. The lipolytic cleavage mechanism leading to lipid (triglyceride) antimicrobial activity, in vivo, was first observed by Canas-Rodriguez and Smith. (a) Canas-Rodriguez, A.; Smith, W. Biochem. J. 1966, 100, 79-82. The identification of the antimicrobial factors of the stomach contents of suckling rabbits; (b) Smith, H. J. Pathol. Bacteriol. 1966, 91, 1-9. The antimicrobial activity of the stomach contents of suckling rabbits. Discussed in: Isaacs, C.E. et al. J. Infect. Dis. 1986, 154, 966. Membrane disruptive effect of human milk: inactivation of enveloped viruses.
29. Isaacs, C.E. et al. Arch. Dis. Childhood 1990, 65, 861. Antiviral and antibacterial lipids in human-milk and infant formula feeds.
30. Thormar, H.; Isaacs, C.E.; Brown, H.R.; Barhatzsky, M.R. Antimicrob. Agents and Chemo. 1987, 31, 27. Inactivation of enveloped viruses and killing of cells by fatty acids and monoglycerides.
31. Kabara, J.J. et al. Antimicrob. Agents Chemother. 1972, 2, 23. Fatty acids and derivatives as antimicrobial agents.
32. Kabara, J.J. et al. Lipids 1977, 12, 753. Antimicrobial lipids: Natural and synthetic fatty acids and monoglycerides.
33. Kabara, J.J. J. Soc. Cosmet. Chem. 1978, 29, 733. Structure-function relationships of surfactants as antimicrobial agents.
34. Kabara, J.J. Nutr. Rev. 1980, 38, 65. Lipids as host-resistance factors of human milk.
35. Kabara, J.J. J. Am. Oil Chem. Soc. 1984, 61, 397. Antimicrobial agents derived from fatty acids.
36. Isaacs, C.E. Thormar, H. 1991. The role of milk-derived antimicrobial lipids as antiviral and antibacterial agents. In: Advances in experimental medicine and biology: immunology of milk and the neonate. Mestecky, J.; Ogra, P.L., eds. New York, NY: Plenum Press, pp. 159-165.
37. Isaacs, C.E. et al. J. Nutr. Biochem. 1992, 3, 304. Addition of lipases to infant formulas produces antiviral and antibacterial activity.
38. Isaacs, C.E. et al. Nutr. Biochem. 1995, 6, 362. Antimicrobial activity of lipids added to human milk, infant formula, and bovine milk.
39. Decuypere, J.A.; Dierick, N.A. Nutr. Res. Rev. 2003, 16, 193-209. The combined use of triacylglycerols containing medium-chain fatty acids and exogenous lipolytic enzymes as an alternative to in-feed antibiotics in piglets: concept, possibilities and limitations. An overview.
40. Bergsson, G. et al. APMIS 2001, 109, 670. Killing of Gram-positive cocci by fatty acids and monoglycerides.
41. Kelsey, J.A.; Bayles, K.W.; Shafii, B.; McGuire, M.A. Lipids 2006, 41, 951. Fatty acids and monoacylglycerols inhibit growth of Staphylococcus aureus.
42. Petra, Š. et al. Eur. J. Lipid Sci. Technol. 2014, 116, 448. Formulation, antibacterial activity, and cytotoxicity of 1-monoacylglycerol microemulsions.
43. Zhang, H. et al. Int. J. Food Microbiol. 2009, 135, 211. Antimicrobial activity of a food-grade fully dilutable microemulsion against Escherichia coli and Staphylococcus aureus.
44. Zhang, H. et al. Int. J. Pharm. 2010, 395, 154. Characterization and antimicrobial activity of a pharmaceutical microemulsion.
45. Fu, X. et al. Int. J. Pharm. 2006, 321, 171. Physicochemical characterization and evaluation of a microemulsion system for antimicrobial activity of glycerol monolaurate.
46. Fu, X. et al. J. Food Process Eng. 2009, 32, 104. Enhancement of antimicrobial activities by the food-grade monolaurin microemulsion system.
47. Thormar, H. et al. App. Environ. Microbiol. 2006, 72, 522. Stable concentrated emulsions of 1-monoglyceride of capric acid (monocaprin) with microbicidal activities against the foodborne bacteria Campylobacter jejuni, Salmonella spp., and Escherichia coli.
48. Bergsson, G. et al. Antimicrob. Agents Chemother. 2002, 20, 258. Bactericidal effects of fatty acids and monoglycerides on Helicobacterpylori.
49. Hilmarrson, H.; Thormar, H.; Thrainsson, J.H.; Gunnarsson, E. Poultry Sci. 2006, 85, 588. Effect of glycerol monocaprate (monocaprin) on broiler chickens: An attempt at reducing intestinal Campylobacter infection.
50. Aurousseau, B.; Thivend, P.; Vermorel, M. Annales de Zootechnique 1984, 33, 219-234. Influence of replacement of part of the tallow in a food by tricaproqne or tricapryline in association with coconut oil on the growth of young preruminant calves.
51. Diereck, N. et al. Livestock Prod. Sci. 2002, 75, 129-142. The combined use of triacylglycerols (TAGs) containing midchain fatty acids (MCFAs) and exogenous lipolytic enzymes as an alternative for nutritional antibiotics in piglet nutrition. I: In vitro screening of the release of MCFAs from selected fat sources by selected exogenous lipolytic enzymes in simulated pig gastric conditions and their effects on the gut flora of piglets.
52. Diereck, N. et al. Livestock Prod. Sci. 2002, 76, 1-16. The combined use of triacylglycerols (TAGs) containing midchain fatty acids (MCFAs) and exogenous lipolytic enzymes as an alternative for nutritional antibiotics in piglet nutrition. II: In vivo release of MCFAs in gastric cannulated and slaughtered piglets by endogenous and exogenous lipases; effects on the luminal gut flora and growth performance.
53. Bergsson, G. et al. Antimicrob. Agents Chemother. 1998, 42, 2290. In vitro inactivation of Chlamydia trachomatis by fatty acids and monoglycerides.
54. Lampe, M.F. et al. Antimicrob. Agents Chemother. 1998, 42, 1239. Killing of Chlamydia trachomatis by novel antimicrobial lipids adapted from compounds of human breast milk.
To view this issue and all back issues online, please visit www.drug-dev.com.
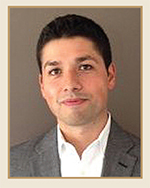
Dr. Ryan Littich is Innovation & Special Programs Manager at ABITEC Corporation. He is responsible for the development of solution-driven lipid excipient technology and applications. Professionally, he draws on experience within a VC-backed biotechnology start-up, having led new product development in an organization commercializing Nobel Prize-winning catalyst technology to transform natural triglycerides into fine and specialty chemicals. He is a classically trained synthetic organic chemist with a foundation in the de novo synthesis of medicinally relevant, complex natural products. He earned his BA in Chemistry from Ferris State University, and his PhD in Synthetic Organic Chemistry from the University of Texas at Austin.
Total Page Views: 4074