Issue:June 2016
PROTEIN CRYSTALS - Reshaping Traditional Biotherapeutic Formulations
INTRODUCTION
Throughout the past decade, protein-based therapeutics have emerged as the key driver of growth in the pharmaceutical industry. R&D pipelines have filled with more and more biologics and, in recent years, monoclonal antibodies have become the fastest growing segment of biological drugs around the world. Despite the success of this segment, there are specific challenges to overcome when developing these types of therapeutics. Unlike small molecules, protein-based therapeutics are almost exclusively administered by parenteral routes.1 Because of the size, biochemical complexity, and low bioavailability of these macromolecules, high doses must also be administered. At high concentrations, protein-protein interactions significantly increase solution viscosities and may result in the formation of aggregates. This in turn decreases manufacturability and complicates drug delivery.2,3 Moreover, aggregates are of special concern because they have been shown to be associated with altered biological activity and increased immunogenicity.4-7 Currently, most protein-based biologics are administered via larger volume, lower concentration formulations via intravenous (IV) infusion. However, these procedures are less patient-friendly, more costly, require trained medical professionals, and often involve a visit to a clinic.
OVERCOMING CHALLENGES WITH CRYSTAL FORMULATIONS
From a cost and patient compliance perspective, subcutaneous injection (SCI) would be the preferred route of administration. To keep patient discomfort to a minimum, SCI volumes generally do not exceed 2 milliliters. The high dose necessary for clinical benefit raises concerns about the exponential relationship between protein concentration and viscosity.8 High viscosity levels reduce syringeability (loading) and injectability (delivery to patient). It has been demonstrated that highly concentrated crystalline suspensions do not result in a similar increase in viscosity (Figure 1).
The viscosity of a suspension (η) is primarily determined by viscosity of the formulation vehicle (η0) and the suspension viscosity is dependent on the crystal volume fraction (Φ) (Figure 2). Because protein crystals are highly organized, tightly packed structures (Figure 3), their volume fraction is considerably less than an equivalent number of protein molecules in solution. The advantages of protein crystals aren’t just limited to lower viscosity.
Proteins are complex macromolecules that require a specific three-dimensional structure in order to be biologically active. The interactions that drive and stabilize higher order secondary, tertiary, and quaternary structures are inherently weak and mainly driven by hydrophobic interactions but also stabilized by hydrogen bonds, salt bridges, and disulfide bonds. As a result, proteins are susceptible to physical or conformational degradation. A number of external factors can cause physical degradation, including higher temperatures, pH, mechanical agitation, and high shear forces to name a few. Crystals present a uniquely stable form of proteins and help protect against many forms of degradation. Moreover, studies have shown that the crystallization process does not affect the biological activity of a protein (Figure 4).
Crystal formulations have long been used for long-acting versions of small molecule drugs. Crystal formulations of therapeutic proteins can also be used to develop products with extended-release properties. Crystal Infliximab administered monthly has the same effect as the soluble form administered weekly in a TNF-α mouse model (Figure 5).
Due to improved handling, increased stability and the possibility of controlled release, crystal formulations of small molecule therapeutics have been on the market for decades.9 However, to date, insulin is the only biologic available in a crystal formulation. What then are the key issues that are preventing the widespread development of these advantageous formulations?
CHALLENGES OF DEVELOPING A CRYSTAL SUSPENSION FORMULATION
There are two main challenges to developing a crystal suspension formulation. The first is to the find a robust crystallization condition that will produce crystals within a short period of time – sufficiently short for GMP manufacturing, preferably less than 24 hours. The second challenge is the development of a drug product formulation that is suitable for injection while maintaining stability in the crystal structure. Finding conditions in which a protein crystallizes is the initial challenge, and oftentimes those crystallization conditions have properties that are not suitable for introduction into patients, ie, non-Generally Regarded As Safe (GRAS) excipients, not isotonic, etc. The second, and often more difficult challenge is then to reformulate the crystal suspension into excipients suitable for injection that also maintain crystal integrity and molecular function upon dissolution.
GMP MANUFACTURING OF THERAPEUTIC CRYSTAL FORMULATIONS
Proteins have been crystallized for structural studies in biochemistry laboratories for over 50 years. However, the requirements of and methods for producing protein crystals for therapeutic purposes are significantly different. Most crystallographers want a single large size crystal (> mm) for structure studies, whereas formulation scientists want very high concentrations of uniform crystals (> 200 mg/mL) that are several orders of magnitude smaller, typically 5 to 30 μm. Protein aggregation is a concern during crystallization – if precipitant amounts are too high, then the individual protein molecules assemble too rapidly and not in order resulting in aggregation. A lot of time and effort is invested in finding the optimal balance to regulate crystal assembly and growth without aggregation.
In addition, the ideal crystallization conditions change as the project moves from vapor diffusion screening to microbatch screening. Conditions that work at the 3-μl level usually don’t translate well to the 15-μl level. Scaling the volume of the crystallization reaction affects how the crystals form. In early development (volumes < 3 μL), evaporation is the primary driver for crystallization. As water evaporates from the small drop, the concentration of excipients increases until crystals form (if conditions are right). In larger volume reactions (> 15 μL and up), there is insufficient surface area for evaporation to be the main driving force behind crystallization. By this point, however, optimization efforts likely have determined the conditions that don’t rely on evaporation to produce crystals with the desired properties. To scale further, it is necessary to move into tank systems (50 mL and up). Tank systems, because of their significantly larger volumes, introduce additional variables that can affect crystal yields and quality; these variables include mixing rate, impeller design, order of excipient addition, and temperature.
Following the discovery of the optimal crystallization conditions, the next step is formulation development. This can be as challenging as developing the optimal crystallization process. Even when a robust process to make small (10 mL) batches of uniform crystals has been developed, the excipients are typically not GRAS. Often, the protein crystals need to be reformulated into GRAS excipients suitable for subcutaneous injection that are also in the desired pH, osmolality range, and break loose energy (BLE – how much force is needed to expel the material from a syringe).
Downstream purification of the desired crystal size can be a challenge. Even with the tightest controls, in each batch, there will be distribution of various crystal size populations. Centrifugation can be used to purify; however, it is not a preferred method. Centrifuge bottles can shed particles thus contaminating the crystals. It’s possible to pre-clean and irradiate the bottles prior to centrifugation, but this adds additional steps to the process. In addition, operators have to manually handle and pour to/from the bottles, introducing risk of spills, errors, and contamination. A better option would be an automated, closed system such as tangential flow filtration (TFF). It reduces the chance for human error, is gentler, and reduces the risk of contamination when compared to centrifugation.
The next steps in the process are fill finish manufacturing, release testing, and visual inspection. There are two major areas of concern when filling crystalline therapeutics: suspension uniformity and fill weight accuracy. In addition to the typical release assays for a protein-based biologic, it’s also important to perform extensive dissolution and biophysical characterization studies of the API pre- and post-crystallization to show protein isn’t affected by the crystallization process or the crystals themselves. The final step is manual visual inspection. Manual visual inspection is the standard in both the US and Europe and heavily relies on the experience, training, and skill of the operator. Specialized training is needed to identify the potential defects in opaque crystalline suspensions that resemble a milky fluid (Figure 6).
THE SOLUTION – ALTHEA’S CRYSTALOMICS® FORMULATION TECHNOLOGY
To help clients develop crystalline formulations, Althea offers access to a proprietary Crystalomics® Formulation Technology. Althea’s unique portfolio of intellectual property encompasses crystallization, cross-linking, and complexation of proteins for therapeutic use. It includes patents, proprietary knowledge, and expertise to develop ideal crystallization conditions, stable crystalline formulations, and scale-up for GMP manufacturing of crystalline suspension drug products. The technology allows companies to produce highly concentrated formulations with low viscosity, enabling low-volume doses and increased stability. The resulting crystalline suspensions are easier to administer and offer the chance to extend the patent life of high-value biologic drugs. A typical crystallization workflow conducted at Althea is shown in Figure 7.
Althea has been successful developing crystallization conditions and stable crystal suspension formulations for over 100 molecules, including antibodies, hormones, enzymes, and peptides from human, animal, and microbial sources.
SUMMARY
While protein therapeutics have enjoyed considerable commercial success throughout the past 3 decades, there still remain formulation and delivery challenges. Due to poor bioavailability and unfavorable pharmacokinetics, frequent administration of large doses is often necessary for clinical benefit. Highly concentrated solutions usually have high viscosity resulting in poor syringeability and injectability. Out of necessity, these products are formulated as low concentration solutions that have to be administered as large volume IV infusions. IV infusions are more expensive, time-consuming, and have to be administered by trained medical professionals. Protein crystals have shown potential to address these issues and can benefit both pharmaceutical developers and patients (Table 1).
REFERENCES
1. Vugmeyster et al. Pharmacokinetics and toxicology of therapeutic proteins: Advances and challenges. World J Biol Chem. 2012 Apr 26;3(4):73-92.
2. Child J. Minireview: Protein Interactions. University of New Hampshire Scholars’ Repository, Fall 2012.
3. Palm et al. The Importance of the Concentration-Temperature-Viscosity Relationship for the Development of Biologics. BioProcess International. Mar. 2015. http://www.bioprocessintl.com/manufacturing/antibody-non-antibody/importanceconcentration-temperature-viscosityrelationship-development-biologics/.
4. Patel et al. Stability Considerations for Biopharmaceuticals, Part 1: Overview of Protein and Peptide Degradation Pathways. BioProcess International. Jan. 2011. http://www.bioprocessintl.com/manufacturing/formulation/stability-considerationsfor-biopharmaceuticals-part-1-332821/.
5. Particle Sciences, Inc. Protein Structure. Technical Brief 2009, Vol. 8. http://www.particlesciences.com/news/technical-briefs/2009/proteinstructure.html.
6. Watts A. (University of Bath), Biological Drugs – Practical Considerations for Handling and Storage. Presentation, May 2013.
7. Kashi R. (Merck Research Laboratory), Challenges in the Development of Stable Protein Formulations for Lung Delivery. Presentation, AAPS Symposium, Sep. 2011.
8. Skalko-Basnet N. Biologics: the role of delivery systems in improved therapy. Biologics Target Ther. 2014;8:107-114.
9. Basu et al. Protein crystals for the delivery of biopharmaceuticals. Expert Opinion Biological Ther. 2004;4(3):301-317.
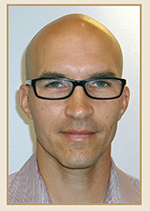
Don Paul Kovarcik is the Technical Marketing Specialist at Ajinomoto Althea, Inc. He is responsible for developing technical marketing pieces for all aspects of Althea’s business, including drug product (fill finish) manufacturing, drug substance manufacturing, Crystalomics® Formulation Technology, and Corynex® Protein Expression System. Prior to joining Althea, he worked in a variety of marketing and business development roles at Lonza in their research products and cell therapy contract manufacturing business units; specifically focused on the development of pluripotent stem cell product and service offerings. He earned his BS in Biochemistry from Virginia Tech and his MBA from Carnegie Mellon University.
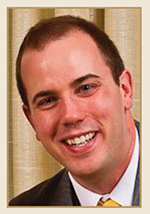
William Wittbold is the Manager for Crystalomics® Technology Transfer at Ajinomoto Althea, Inc. After earning his BS and MS in Microbiology from the University of Massachusetts Amherst, he worked in positions with InfiMed Therapeutics, University of Massachusetts Medical School, Altus Pharmaceuticals and Wyatt Technology. With an extensive background in protein crystallization, biophysical characterization, and assay development, he guides client and internal projects from screening through GMP manufacturing and fill finish. He has diverse experience working with clients ranging from startups to large pharmaceutical companies.
Total Page Views: 6740