Issue:October 2016
TRAINING DEVICES - Best Practices & Considerations in Developing Effective Training Devices for Injectable Healthcare Markets
INTRODUCTION
Over the years, many industry stakeholders and pharmaceutical manufacturers have come to realize the importance of training and the role it has on promoting healthy patient outcomes and effective disease management. Many studies suggest that without proper training during the onboarding process, or the first 30 to 90 days of treatment, patients are more likely to drop off from therapy or incorrectly use drug delivery devices, such as autoinjectors, prefilled syringes, and other forms of self-administration.
One of the most common drug delivery devices on the market currently is the autoinjector. Designed primarily for patient at-home use, autoinjectors were developed to improve the patient experience and address the limitations and administration barriers of legacy injection systems. Many of today’s autoinjectors incorporate tactile and mechanical features that provide auditory and visual feedback during the injection process.2 While these devices provide a number of benefits to patients, administration errors and device misuse can result in injuries and sub-optimal therapeutic outcomes for patients.
According to a study conducted by the University of Texas Medical Branch at Galveston (UTMB), 84% of patients failed to demonstrate correct use of an autoinjector, with more than half skipping up to four steps. Common errors included: failing to hold the device correctly, choosing an unsuitable injection site, and not applying enough force to actuate the drug delivery device. The study also found that, at 86%, “wet injections” were the most common patient error, which in practice would result in patients not receiving their full prescribed doses of medicine.1
Further research demonstrates that many patients do not read or fully understand the instructions for use (IFU) that accompany their drug delivery device. A study conducted by Noble and researchers from Auburn University surveyed more than 700 injection experienced patients and found that more half did not read their IFU document prior to beginning treatment.
In addition to traditional instructions and package inserts, healthcare providers are often leveraged as learned intermediaries to onboard patients and provide access to training and education. While these training strategies can be very effective, research suggests that there is often a great deal of variability and inconsistencies with these methods of trainings and patients’ ability to retain this information and apply it to the successful use of their delivery system.
To address the common gaps in patient onboarding, training devices are often used to create consistent onboarding experiences for patients through the use of novel technologies and mechanisms that fully simulate the mechanical aspects of the injection experience. While these devices appear to be fairly simple at first glance, numerous design and engineering challenges must be addressed in order to successfully develop training devices and other onboarding solutions.
INTERNAL DESIGN & TECHNOLOGY OF TRAINING DEVICES
Engineering training devices for manufacturability and repeatability is a delicate balance. Fully understanding device development and mechanical design is one of the first steps in engineering robust training device solutions. The exterior of the device should emulate the real autoinjector so that patients become familiar with key features and physical characteristics such as the look, feel, and weight of their commercial delivery devices. The interior design of training devices also need to be meticulously engineered in order to provide a proper training experience. To accomplish this, human factors are taken into consideration throughout the design process to ensure that training devices align with the physical, cognitive, and emotional needs of users. In addition to understanding user needs, Noble has analyzed a variety of on-market delivery systems to understand their handling requirements and critical functions. Though in some cases mechanisms similar to commercial devices are used, ground-up mechanical design is usually employed to integrate all necessary functions in a resettable and reusable training device. This means that the trainer will look the same on the outside; however, internally it will be vastly different.
Because one of the most common autoinjector errors is the aforementioned “wet injection,” accurately simulating plunger speed is a key component to designing an effective training device that can be used to properly onboard patients. This is done by setting target ranges based on the specifications of a commercial product to ensure that the training device replicates the plunger drop speed that is representative of patients’ actual injections. Plunger simulation also takes into account the formulation characteristics of therapeutic entities, such as the viscosity and dosing volume, without containing an actual liquid. This is done so that patients can realistically learn how long it takes for the medication to deploy by tracking the progress through the end-of-dose indicator viewing window.
Many autoinjectors utilize a stored-energy feature, typically a compression spring, to energize the power-pack and deploy the plunger. Repeating this design within a trainer can be very challenging because the inherent viscous damping in the medicament delivery device does not exist in the trainer, as training devices do not contain needles or liquid. Therefore, to accurately represent plunger speeds, controllable friction and damping must be created with alternative methods. Significant research and development efforts have gone into this challenge, especially considering the trend of higher dosing volumes and increased injection times, some of which now target 15 or more seconds.
In addition to addressing speed control, all functions that require force application by the user must accurately represent the real device. These include cap removal force, safety shield deflection force, and activation force (for shield or button-activated). Force profiles can also play a significant role; some forces may ramp-up slowly while others have a fast on-set for activation. There are also other unique devices that require unlock steps, twisting, priming, shaking, and a variety of other features to replicate. Other considerations include representing the audible click levels that are present and integrating tactile feel elements, such as subtle internal mechanism vibrations. All these items included, along with the fact that it needs to be easily resettable versus just a single-use product, the trainer must also maintain a 1:1 size ratio (ie, it cannot get any larger than the real drug delivery device).
EXTERNAL DESIGN OF THE TRAINING DEVICE
As mentioned previously, external details are also crucial to the design and engineering process. Characteristics of the autoinjector such as the shape, barrel dimensions, viewing window, size, and shape of the actuation button, needle shield, and end cap are all accurately matched so that patients are able to familiarize themselves with the look and feel of the device. However, this is complex because the interior of a training device contains additional mechanisms that allow the device to be used multiple times.
One of the most seemingly simple design challenges is to make the device look like the real product externally. Upon further investigation though, this can present its own challenges. For example, if the trainer looks exactly like the real device, one may mistakenly use a trainer in an emergency or vice-versa. This is typically addressed with optimized packaging, labeling, and graphical training instructions. Trainers usually have large labels that read “Trainer, This Device Contains No Needles or Drug.” Though in every other regard, the trainer appears exactly the same: size, shape, textures, and Pantone-matched color schemes (or complementary colors to denote that it is a trainer).
Other considerations that must be prioritized are ancillary training features like augmented auditory or video-based training instructions. Many of the trainers currently in development include some form of collateral training like talking packaging, sensor-based error-correction, smart device application, or a combination of these features.
QUALITY CONTROL PROCESS
Quality design standards are paramount when designing training devices in order to ensure that every patient has a consistent and accurate training experience. Noble conducts rigorous device testing, taking into consideration each brand’s specified requirements. One of the keys to success is utilizing optimized Standard Operating Procedures (SOPs) and Standard Inspection Procedures (SIPs) in the assembly process at the factory. Many manual and semi-automated tests and inspections are integrated throughout the process to verify targets will be met on the final assembly stage, reducing scrap rate and ensuring a high-quality product.
Critical functions like shield and activation forces are tested at several points during assembly. Plunger speed, one of the most important characteristics, is measured at three different points in the line. The final step in assembly is a 100% inspection and test on all functions, a luxury that can be afforded with a reusable trainer versus a single-use drug delivery device. During pilot runs, many other tests are also performed to determine steady-state value results. Some of these include environmental, accelerated aging/life, shipping, drop-testing, and materials compliance. Though not a formally regulated device category, Noble treats the design and manufacturing of mechanical trainers much like a regulated product to ensure the highest final quality product.
To ensure the autoinjection times and other important functions of device trainers are consistent with those in the actual drug delivery devices, Noble also performs Acceptable Quality Limit (AQL) sampling. For example, Noble recently pulled a random sample of 35 units from the final production line, and each device was tested over a 6-week period. The target range for injection speed requested by the client was 3 to 12 seconds. Not only did the training devices all fall within this range, the devices averaged at approximately 5.51 seconds per week throughout injection time testing, which was within Noble’s more rigorous internal standard of 4 to 7.5 seconds.
CONCLUSION
As training technology becomes more prevalent in the pharmaceutical industry, the engineering and capabilities of these devices will continue to advance, creating a more complex and intricate engineering process. These advancements are necessary as they will allow patients to become more confident in their treatments, overcome treatment barriers, and ultimately lead healthier lives.
In order for training devices to work efficiently, it is necessary that the devices are tested with stringent standards. Patients need to become familiar with the device in order for them to learn and anticipate the steps necessary for proper drug administration. This requires training devices to accurately replicate the ergonomics, interaction, and injection time of the actual device.
In today’s market, a growing number of patients are being prescribed self-administered injectable treatments. Pharmaceutical companies that prioritize the patient experience, using training technology to help these patients properly onboard to therapy, will continue to benefit through competitive advantages and the value they create within the industry.
REFERENCES
1. Bonds RS, Asawa A, Ghazi AI. Misuse of medical devices: a persistent problem in self-management of asthma and allergic disease. Annals of Allergy, Asthma & Immunology. 2014;114(1):74-76e2. doi:10.1016/j.anai.2014.10.016 4. The UTMB Newsroom, UTMB Health, UTMB.edu. 2014. Retrieved from http://www.utmb.edu/newsroom/article10169.aspx.
2. Devices and combination products for biopharmaceuticals. In: Jameel F, Hershenson S, Khan MA, MartinMoe S, Eds. Quality by design for biopharmaceutical drug product development. 2015:414-420. New York, NY: Springer-Verlag.
3. Kessels RP. Patients’ Memory for Medical Information. 2003. Retrieved from http://www.ncbi.nlm.nih.gov/pmc/articles/PMC539473/ 7. Adler M. Challenges in the development of prefilled syringes for biologics from a formulation scientist’s point of view. Am Pharm Rev. 2000. Retrieved from http://www. americanpharmaceuticalreview.com/ Featured-Articles/38372-Challenges-inthe-Development-of-Pre-filled-Syringesfor-Biologics-from-a-FormulationScientist-s-Point-of-View.
4. Sicherer S, Forman J, Noone S. Use assessment of self-administered epinephrine among food-allergic children and pediatricians. PubMed – NCBI. 2000. Retrieved from http://www.ncbi.nlm.nih.gov/pubmed/10654956.
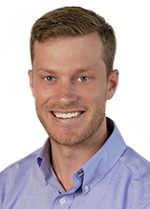
Joe Reynolds is Research Manager at Noble, where he leverages his knowledge and experience to develop and implement strategies that improve the patient experience and maximize value for stakeholders. His experiences include commercial, managed care and product development initiatives with leading medical device, pharmaceutical and biopharmaceutical manufacturers. Mr. Reynolds earned his Bachelor of Science in Business Administration from the University of Central Florida, a Master of Science in Marketing from the University of South Florida, and a Master of Science in Pharmacy and Master Certificate in Drug Regulatory Affairs from the University of Florida.
Total Page Views: 4843