Issue:September 2015
SPRAY-DRIED DISPERSIONS - Developing Process Control Strategies for the Manufacture of Spray-Dried Dispersions
ABSTRACT
A significant share of current drug development pipelines include active pharmaceutical ingredients (APIs) that fall into BCS (biopharmaceutical classification system) 2 and 4 spaces. These compounds are typically characterized by their low-aqueous solubility and thus poor bioavailability, requiring advanced formulation approaches.1 Various approaches have been developed to enable these types of compounds, including particle size reduction (eg, micronization), chemical modification (eg, salt forms and pro-drugs), complexing agents, solubilized liquid forms (eg, SEDDS), and solid amorphous dispersions.
In recent years, amorphous dispersions manufactured via spray drying have gained considerable traction with the commercialization of numerous products. Additionally, increased access to and familiarity with small-scale spray drying equipment has enabled formulation scientists to include spray-dried dispersions (SDDs) early in compound screening and drug development. With greater use of SDDs as an integral part of the formulation toolbox, and a considerable portfolio of SDDs progressing toward commercialization, adoption of a Quality-by-Design (QbD)-based development approach can help streamline scale-up and regulatory approval in bringing these compounds to market.
INTRODUCTION
This paper will broadly describe a model-based SDD product development methodology as well as a control strategy that is based upon fundamental understanding of the formulation and the process. The product development flow and general activities for SDD product development are presented in Figure 1. Key to this development strategy is the early identification of critical SDD attributes and process parameters, which contributes to the development program and facilitates identification of functional relationships between key material attributes and their controlling parameters. This understanding can later be translated into a robust control strategy for commercial manufacture.
IDENTIFYING THE IMPORTANT SDD ATTRIBUTES
SDD development begins with identifying the formulation goals, or Target Product Profile (TPP), of the compound and designing a formulation to achieve those goals. Formulation maps, which consider the API’s physical and chemical properties, can be used in conjunction with the TPP to identify a formulation that is likely to produce a physically stable SDD with the desired performance early in development.2 The TPP that is created during initial formulation screening can guide the entire development process and be refined with additional product and process information.
Early in a development program, predictive in vitro dissolution tests and physical stability maps can be combined with risk assessments to identify critical quality attributes (CQAs) for further study. Building a mechanistic understanding of the in vitro dissolution of an SDD guides thinking in the early stages of product development and ultimately allows prediction of the impact of SDD attributes on in vivo performance. Similarly, physical stability maps can be used to predict whether drug loading, humidity, residual solvent levels, or temperature exposure represent a risk to the stability of the SDD during manufacture or long-term storage.
Several common CQAs and their potential impact on the TPP are listed in Table 1. The formulation and process parameters that commonly define the SDD attributes are also shown in Table 1.
DETERMINING THE IMPORTANT SPRAY DRYING PARAMETERS
Spray drying is a well understood process that is ideally suited for the manufacture of amorphous dispersions. As shown in Figure 2, drug and polymer are dissolved in an organic solvent to form a solution that is atomized into droplets. The droplets are dried upon contact with a hot drying gas inside the spray drying chamber. Residual solvent remaining on the SDD after spray drying is removed in a secondary drying step. In this paper, we will focus on the spray drying unit operation and will not discuss solution preparation or secondary drying operations. As described in previous articles contributed by Bend Research, the process parameters required to produce target SDD attributes can be defined and controlled by understanding two key aspects of spray drying: droplet formation or atomization and droplet drying.3 Droplet size and drying rate parameters can be correlated to SDD attributes.
UNDERSTANDING & ESTABLISHING FUNCTIONAL RELATIONSHIPS
Dependence of the critical SDD attributes on formulation and process parameters can be understood through investigation of the formulation material attributes and the spray drying process.
Material Attribute Evaluation – Solid State
For an amorphous dispersion, a fundamental understanding and study of the solid state during manufacture and upon storage is critical to controlling and maintaining physical stability. Physical stability of a spray dried amorphous dispersion is governed thermodynamically by the miscibility of the drug and polymer and kinetically by molecular mobility. Primary physical stability failure modes include phase separation and/or crystallization. The mobility of a SDD is dependent on the formulation through the glass transition temperatures (Tg) of the components, and on the degree of plasticization from residual solvents or water.4,5 The impact of humidity can be assessed by equilibrating samples to a range of relative humidity (%RH) conditions and measuring the Tg of the humidified SDD sample. This data can be used to predict the long-term stability of an SDD at a given storage condition and to establish functional relationships between SDD attributes and storage parameters, including temperature, RH, time, and packaging configuration.
Analogous to the situation with water, residual spray solvent plasticizes a SDD and lowers the Tg, sometimes significantly.6 Understanding the impact of residual solvent on the Tg is critical to ensure robust manufacturing and in-process stability. This effect can be estimated by the Fox equation and studied with solvent vapor sorption and calorimetry experiments, as seen in Figures 3 and 4. The spray drying process can be defined by the relative saturation (%RS) of solvent inside the dryer, which is a function of the key spray drying parameters. Common ranges of dryer outlet %RS may vary between 10% and 35% depending on the solvent and the spray drying parameters. The residual solvent remaining on the SDD after spray drying can be predicted by calculating the %RS from the spray drying parameters and conducting a vapor sorption study on the SDD, as shown in Figure 3. The Tg of the solvent wet SDD can be predicted, as shown in Figure 4. This data can be used to select spray drying parameters to yield spray dryer outlet target %RS and SDD solvent content. Quantifying the dependence of a formulation’s physical stability and residual solvent on spray drying, secondary drying, and storage parameters is critical to selecting the appropriate operating space, storage conditions, and packaging controls.
Material Attribute Evaluation – Dissolution
In addition to studying the physical state of the SDD, understanding the SDD performance mechanism by analyzing dissolution can help define attribute and parameter criticality and functionality. SDD dissolution mechanisms vary depending on the API physical and chemical properties and the specific formulation, but the mechanism for most SDDs can be described by two scenarios: erosion and spinodal decomposition.7 Most SDD dissolution mechanisms are a combination of these scenarios, and fall on a spectrum between the two. In both mechanisms, water diffuses into the SDD particle, and the polymer hydrates. If the drug is largely soluble in the hydrated polymer, the particle erodes at the edges, and the drug dissolves and diffuses away from the particle at the boundary layer. In the second case, if the drug is largely insoluble in the hydrated polymer, drug-rich amorphous domains phase separate and are stabilized as nanoparticles through an added surfactant or amphiphilic and charged polymers, leading to disintegration of the primary SDD particle into drug/polymer nanoparticles.
By performing tests at non-sink conditions in biologically relevant dissolution media, it is possible to measure the dissolution rate and extent of supersaturation that SDDs provide relative to the crystalline API. Differential centrifugation, light scattering, UV-Vis spectroscopy, and other techniques help to identify and measure the formation of drug-related species important to absorption in vivo, and yield insight into the dissolution mechanism.
In a SDD formulation in which the dissolution mechanism more closely follows the erosion-based model, the dissolution rate is often inversely related to the particle size of the SDD powder. Maximizing the surface area of the powder by making smaller particles can improve the dissolution rate of the drug. As shown in Figure 5, the particle size has potential to significantly impact the dissolution performance of a SDD. Understanding the important material attributes with these in vitro dissolution tests can define target SDD particle size targets and spray drying parameters.
Spray Drying Process Parameters – Atomization
The droplet size, coupled with the solid components and their solubility, ultimately define the SDD particle size. As previously described, particle size can be an SDD CQA if it impacts in vivo dissolution. The interdependent spray drying parameters that define droplet size are the nozzle geometry, spray solution properties (viscosity, density, and surface tension), solution flow rate, and nozzle pressure. Droplet size can be established both by direct measurement and/or by empirical correlations for a specific nozzle geometry. Droplet size can be correlated to particle size through range-finding experiments and formal Design of Experiments (DOEs). After identifying the target SDD particle size that meets dissolution requirements, the upper droplet size can be constrained and associated nozzle geometries and atomization conditions identified.
Spray Drying Process Parameters – Drying Rate
Upon fixing the target droplet size, the droplet drying rate is defined by the thermodynamics of the system, which can be predicted and characterized by a simple mass and energy balance on the spray dryer. The outlet temperature and calculated %RS characterize kinetic- and equilibrium-driving forces for evaporation of the solvent from the droplets and correlate with potentially critical SDD attributes such as bulk density, residual solvent and physical state. The material attribute studies described above can be used to select low-risk manufacturing conditions. These correlations of dryer outlet temperature (or %RS) to the SDD attributes are established through range finding and screening DOEs. A wide droplet size and drying rate space can be evaluated to develop correlations and establish a wide acceptable SDD attribute space. This space needs to consider the downstream process space, as both bulk density and particle size may be critical inputs for the selected dosage form.8 The correlations in conjunction with continued risk assessments can be used to define attribute and parameter criticality.
CONTROLLING THE CRITICAL PARAMETERS
Once SDD CQAs are identified, a robust control strategy for the critical process parameters (CPPs) can be implemented by maintaining target droplet size and drying rate.
To ensure robust control over droplet size and thus SDD particle sizes, the nozzle geometry and operating conditions are selected with inherent stability. Figure 6 shows a graph of droplet size verses nozzle pressure for a specific nozzle geometry. The target droplet size can be maintained over a wide range of nozzle pressures for this nozzle and formulation. Therefore, slight variations in nozzle pressure will produce the same target droplet size and SDD particle size. Under this control strategy, nozzle pressure becomes the critical control parameter for ensuring that the target droplet size is maintained, as shown in Figure 6.
Once droplet size is fixed, the spray dryer thermodynamics can be used to ensure robust control over drying rate and thus SDD bulk density, physical state, and residual solvent. The key interdependent thermodynamic process parameters are dryer outlet temperature, solution flow rate, drying gas inlet temperature, drying gas flow rate, and condenser temperature, which dictates the amount of solvent vapor recycled into the inlet gas stream.9 These parameters are all related through mass and energy balances. Maintaining dryer outlet temperature within the proven acceptable range ensures that the target SDD attributes are achieved. Drying gas is typically fixed over a narrow range for a given scale spray dryer. Condenser temperature is easily maintained within a narrow range and is limited by chilling equipment. Solution flow rate may vary slightly within a predetermined range to maintain atomization parameters and target droplet size.
Therefore, drying gas inlet temperature is varied within a predetermined and calculable range to maintain target outlet temperature and SDD attributes, as shown in Figure 7. In addition, the interdependence of the thermodynamic parameters through the mass and energy balance can be used to demonstrate a state of process control.
SUMMARY
Drug pipelines increasingly feature new drug candidates that exhibit poor solubility and require well-established enabling technologies to address this critical issue. In addition to being an enabling technology for low-solubility compounds, spray drying has a track-record of success for robust processing and correspondingly strong commercializable control strategies. In development, CQAs are rapidly identified to achieve the target product profile. Through fundamental, semi-empirical, and/or empirical modeling, CQAs are related to the CPPs that determine them. Knowledge of these relationships and mechanistic understanding of performance and physical stability lead to the selection of processing parameter ranges that maintain CQA target ranges. This is the foundation of both a strong regulatory submission and a control strategy to deliver successful commercial products.
REFERENCES
1. Zografi G, Newman A. Introduction to amorphous solid dispersion. Pharmaceutical Amorphous Solid Dispersions. Wiley Publishing, Hoboken, NJ, March 2015.
2. Bloom CJ, Lyon DK. Amorphous dispersions & other tools for drug discovery formulation support. Drug Development & Delivery. 2013;13(3):32-37.
3. DuBose D, Settell D, Baumann J. Efficient scale-up strategy for spray-dried dispersions. Drug Development & Delivery. 2013;13(8):54-62.
4. Gordon M, Taylor JS. Ideal copolymers and the second-order transitions of synthetic rubbers. i. non-crystalline copolymers. Journal of Applied Chemistry 2. 1952:493-500.
5. Fox TG. Bulletin of the Journal of American Physical Society 1;1956:123.
6. Gil M, Vicente J, Gaspar F. Scale-up methodology for pharmaceutical spray-drying. Chemistry Today. 2010;10(4):18-22.
7. Vodak D, Morgen M. Design and development of HPMCAS-based spray-dried dispersions. Amorphous Solid Dispersions: Theory and Practice 1. Wiley Publishing, Hoboken, NJ. 2014:303-322.
8. Demuth B, Nagy ZK, et. Al. Downstream processing of polymer-based amorphous solid dispersions to generate tablet formulations. International Journal of Pharmaceutics. 2015;486:268-286.
9. Dobry D, Settell D, Baumann JM, Ray R, Graham L, Beyerinck R. A model-based methodology for spray-drying process development. Journal of Pharmaceutical Innovation 4. 2009:133-142.
To view this issue and all back issues online, please visit www.drugdev.com.
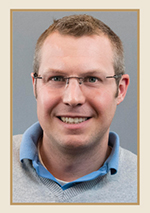
Devon DuBose is a Director of Engineering at Bend Research, a division of Capsugel Dosage Form Solutions. His responsibilities include spray drying development and scale-up for preclinical and clinical projects, and transferring spray drying processes into a current Good Manufacturing Practice environment. He also has expertise with special spray drying projects for biologics and inhalation and has led numerous private client programs, ranging from preclinical feasibility through Phase III clinical manufacture. Mr. DuBose has been with Bend Research since 2007. He graduated with a BS in Chemical Engineering from Oregon State University and is a registered professional engineer in the State of Oregon. Mr. DuBose has four U.S. patents pending.
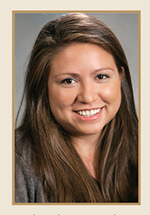
Dana Settell is Vice President at Bend Research, a division of Capsugel Dosage Form Solutions, where she oversees late-stage engineering and manufacturing programs. She has extensive experience with scaling up drug production from development through commercial manufacture. She was instrumental in the startup of a commercial plant plan to manufacture spray-dried dispersion technology at the metric ton scale. Ms. Settell earned a Bachelor’s degree in Chemical Engineering from the University of Colorado. She holds two patents and is a member of the American Institute of Chemical Engineers.
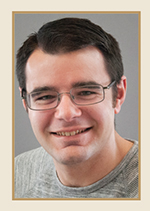
Nathan Bennette is a Senior Research Chemist and Technical Lead at Bend Research, a division of Capsugel Dosage Form Solutions. In collaboration with engineering and manufacturing teams, he leads a team of scientists to develop high-energy solid-state formulations and solid dosage forms for oral delivery. His primary areas of expertise and responsibility include the development, characterization, and scale-up of solid amorphous dispersions and nano-crystalline formulations for bioavailability enhancement. He earned his MS in Inorganic/Organometallic Chemistry from the University of Washington and his BS in Chemistry from the University of Oregon.
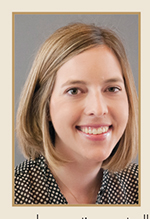
Amber Broadbent, PhD is a Director of Engineering at Bend Research, a division of Capsugel Dosage Form Solutions. She leads interdisciplinary teams of scientists and engineers to develop and manufacture novel pharmaceutical formulations. Her primary areas of expertise include process development, scale-up, and commercialization of spray dried-dispersions (SDDs) and osmotic controlled-release tablets. Dr. Broadbent has been with Bend Research since 2011. She earned her PhD in Engineering, with a Chemical Engineering focus, from Montana State University.
Total Page Views: 11492