Issue:October 2022
PLATFORM TECHNOLOGY - The Evolution of Cancer Vaccines: Moving Beyond Failure & a New Era for Cancer Treatment
INTRODUCTION
Historically, therapeutic vaccines as a viable cancer treatment have had mixed levels of success. Despite strong beginnings with the work of Thiery Boon and his colleagues, who discovered that T cells could recognize proteins from cancerous cells, progress of cancer vaccines diminished after multiple failures using dozens of combinations of antigens, adjuvants, and delivery technologies.1-3
Following Boon’s research, data from clinical trials evaluating therapies targeting long peptides, minimal epitope peptides and proteins have shown mixed results. With limited understanding of the physiological response that the body naturally produces, treatment has been widely systemic and not targeted, resulting in adverse effects and minimal success in outcomes. Immune cells in the lymph nodes have not been activated specifically to mimic the natural immune response. Also, conventional and chemical adjuvants overwhelmed the system, and targeting heterogeneously expressed antigens had limited success, eventually leading to a pause in cancer vaccine development.4,5
Recently, the immuno-oncology landscape has seen a resurgence of vaccines using new methods leveraging the body’s natural immune system. New studies show promising data with the use of mRNA-based vaccines and the injection of nanoparticles into regional lymph nodes to achieve disease stabilization.6 This has led to a potentially groundbreaking era of therapeutic cancer vaccines thanks to discoveries in identifying truncal targets, targeting tumor neoantigens and, notably, developing improved delivery technologies that stimulate a robust, targeted and persistent immune response.
DISCOVERY & CHALLENGE IN DEVELOPING TARGETED CANCER VACCINES
Increased understanding of the immune system and cancer biology has allowed for improved cancer vaccine design. Cancers develop primarily by genetic mutations that result in abnormal and uncontrolled cell growth, driving tumor progression and promoting tumor survival, proliferation, and immune evasion. The successful identification of these “driver mutations” and better understanding of the underlying biological mechanisms enabled the development of more targeted therapies.
Historically, cancer vaccines were developed using peptides derived from tumor-associated antigens (TAAs). TAAs are proteins highly expressed in cancer cells and present on their surface, which allows them to be recognized by T cells during the anti-tumor response.7 However, this approach can be limited in inducing an effective response and can lead to toxicity issues as some of these antigens are also found on healthy cells, presenting a discrimination challenge to the immune system.
Further research to understand the immunosuppressive and resistant nature of tumors and how they can evade immune surveillance led to the emergence of checkpoint inhibitors (CPIs), which have been successfully implemented in combination with targeted therapies. Unfortunately, some tumors have no or limited response to these treatments even in combination, as cancers can evolve to evade immune recognition. These resistance mechanisms are a challenge in immunotherapies today and partly why many treatments have been unsuccessful in clinical trials. Additionally, the tumor microenvironment (TME) itself can further promote cancer survival.8 Chronic inflammation can result from an immunosuppressive TME, further enabling evasion from immune surveillance and promoting cancer progression. A tumor’s inflammatory status can also be a predictor of treatment efficiency. CPI treatments may reverse immune suppression of immune inflamed tumors but do not always work. Non-inflamed tumors or “immune-desert” tumors rarely respond to CPIs.
Another limitation with cancer immunotherapy is that patients often have weakened immune systems from prior treatments such as chemotherapy, which means treatments need to be highly effective at stimulating a sustained and potent anti-tumor immune response. This is especially challenging given that cancer is usually diagnosed in older people, where the immune system is slowly declining via a process known as “immunosenescence.” This process is caused by changes in the lymph nodes impacting the adaptive and innate immune system functions.9
CONSIDERATIONS IN DELIVERY & FORMULATION OF CANCER VACCINES
The addition of immunomodulatory agents such as adjuvants could improve clinical efficacy, especially for antigen targets that are poorly immunogenic. Adjuvants can aid in optimizing immune responses, which can address immunosenescence in elderly patients and cancer patients. In a compromised immune system, with the suppressive TME, adjuvants elicit limited tumor-specific T cell response. Choice of adjuvant must be carefully considered to ensure proper stimulation, potency, and durability of a tumor-specific immune response.
The mechanism of how cancer vaccines are delivered is equally important. Technologies such as viral vectors, oncolytic viruses, and mRNA-based vaccines have all emerged as viable options to facilitate antigen delivery and improve the immune response of vaccine therapies.10 Other aspects to consider are formulations to facilitate improved delivery of antigens, such as water in oil or oil in water. Each of these treatment options has benefits and drawbacks.
Delivering target antigens to the appropriate innate immune cells must also have the right cues for maturation in order to activate specific T cells. Target antigens should be delivered to antigen presenting cells (APCs) which in turn activate specific T cells and other cells of the innate and adaptive immune system, engaging both T and B cells. To better evoke an effective immune response, cancer vaccines must mimic natural biology and train the immune system to fight off the cancer, which is continuously evolving to evade the immune system.11
In cancer vaccine development, researchers need to consider all relevant components for optimal therapeutic efficacy. A vaccine not only needs a precise target to destroy cancer cells and minimize residual disease, but the proper delivery vehicle and adjuvants to induce a long-lasting response in addition to limiting toxicity and adverse events.12-14 New treatments have emerged, leveraging lessons learned and offering a well-rounded approach by combining target antigens with an optimized delivery technology to induce an appropriate and effective immune response.
A DIFFERENTIATED APPROACH THAT COULD USHER IN A NEW ERA OF CANCER TREATMENT
Today, multiple novel cancer treatment approaches are arising, including the DPX® platform developed by IMV Inc. This delivery technology educates a targeted, robust, persistent immune response in patients by mimicking the physiologic condition of exposing the immune system to a target antigen. The DPX platform is a lipid-in-oil technology that has practical advantages compared to standard emulsions or traditional peptide delivery methods (Figure 1).
Compared to standard emulsions that diffuse payload into surrounding tissues over time, the DPX formulation maintains its cargo in a concentrated volume at the site of injection, where it interacts with immune cells for a prolonged time (Figure 2). This prevents diffusion into tissues and bloodstream while reducing side effects. APCs are recruited and actively uptake the bioactive molecules packaged in DPX. APCs then travel to the lymph node where they activate lymphocytes in a targeted fashion. A robust antigen-specific immune response is elicited, involving T cells, B cells, and natural killer (NK) cells. These cells then circulate in the blood, infiltrating tumors, destroying only the cancerous cells. Studies have shown that peptides formulated in DPX consistently generate greater T cell responses than in other formulations.15
DPX-based therapies stimulate the immune system, leveraging the body’s natural mechanisms to fight disease with a comprehensive immune response. These immunotherapies have also shown synergistic effect with checkpoint inhibitors as well as other treatments.
DPX-based therapies can include multiple adjuvants to optimize a prolonged immune response. The DPX platform is versatile and capable of delivering a wide range of bioactive molecules including peptides, proteins, virus-like particles, nucleic acids, mRNA, or other small molecules (Figure 3). One specific drug candidate in development that leverages the DPX platform technology is maveropepimut-S (MVP-S), which incorporates survivin-specific immunogenic peptides to treat cancers. Survivin is a protein commonly expressed in various cancers which promotes aberrant cell growth and contributes to cancer biology. It is a biomarker of poor prognosis and confers resistance to chemotherapy and radiation. Targeting survivin selectively targets only cancer cells and circumvents off-target toxicities, as it is not expressed on normal healthy cells.
Other practical advantages include the delivery path via subcutaneous injection with a simple in-office administration. In addition, DPX-based therapies are fully synthetic and easy to manufacture with long-term stability. Shelf life for DPX-based products can last years compared to aqueous or conventional emulsions, making the supply chain easier to manage. Given the versatility of the platform, this technology can be applicable in infectious diseases.
CLINICAL TRIALS OF MVP-S VALIDATE THE POTENTIAL OF THE DPX PLATFORM
IMV is evaluating DPX-based assets in several clinical trials in difficult-to-treat cancer indications, including diffuse large B-cell lymphoma (DLBCL), ovarian, breast, and bladder cancer. Previous studies have shown encouraging results, notably in patients who have failed on prior lines of therapy. MVP-S is the clinical validation and proof of concept of the DPX platform’s potential in hematologic and solid cancer indications with and without CPIs.
MVP-S has demonstrated a specific anti-tumor immune response along with a favorable safety profile. Recent findings from IMV’s Phase 2 clinical trial, DeCidE1, which examined MVP-S with intermittent low dose cyclophosphamide (CPA) in patients with advanced, recurrent ovarian cancer, suggest that immunogenic tumors are more susceptible to MVP-S treatment. Around 70% of patients diagnosed with ovarian cancer will have a recurrence.16 Notably, most patients in the DeCidE1 study had been heavily pre-treated and specifically 57.9% were platinum resistant but responded to the MVP-S treatment. The trial has proven the mechanism of action of MVP-S elicits a robust immune response that involves T, B, and NK cells. These results support additional clinical studies of MVP-S in ovarian cancer. The company has recently dosed its first patient in the AVALON trial, an open label, multi-center, Phase 2b study evaluating MVP-S with intermittent, low-dose CPA in patients with platinum-resistant ovarian cancer.
Another Phase 2 trial, SPiReL, evaluating MVP-S/CPA and pembrolizumab in subjects with recurrent/refractory DLBCL (r/r DLBCL), has also shown clinical benefit in patients. DLBCL remains the most common non-Hodgkin’s lymphoma with a 5-year survival rate of about 64%. The results of the SPiReL trial specifically showed long duration of clinical benefit in 77.8% of evaluated patients and survivin-specific T cell responses with a favorable safety profile. Building off these promising results, a second open label, multi-center, Phase 2b trial, VITALIZE, is currently being conducted evaluating MVP-S/CPA in combination with pembrolizumab in patients with r/r DLBCL.
Finally, a Phase 2 “basket” trial evaluating MVP-S/CPA in combination with pembrolizumab in multiple cancers showed promising results in tumors positive for the microsatellite instability high biomarker, as well as bladder cancer patients. In metastatic bladder cancer, MVP-S has induced robust survivin-specific T cell responses and was shown to provide clinical benefit in patients who had previously received prior immune checkpoint inhibitor therapy, making MVP-S a potentially promising treatment option.
In conclusion, MVP-S offers many unique and advantageous properties to educate the immune system and promote a robust, targeted, and persistent immune response. DPX-based therapies, such as MVP-S, uniquely mimic the physiologic condition of exposing the immune system to a target antigen. As a novel immune educating platform, DPX offers:
- Targeted and physiologic delivery of payload to lymph nodes
- Effective antigen presentation and uptake
- Robust T cell activation and innate immune stimulation and recruitment
- Potentially unprecedented clinical datasets
- Versatility to deliver a wide range of cargo
- Plug-and–play platform that can be leveraged across oncology, and across therapeutic areas to deliver appropriate cues to the immune system
Despite the many challenges researchers have faced in identifying effective, targeted, non-toxic cancer vaccine treatments, guided by the historical findings and advancements made in the therapeutic cancer vaccine landscape, the DPX platform has shown promising potential for cancer treatment and offers a unique approach in immunotherapy to elicit a targeted immune response and bolster patient quality of life.
REFERENCES
- van der Bruggen, P., Traversari, C., Chomez, P., Lurquin, C., De Plaen, E., Van den Eynde, B., Knuth, A., & Boon, T. (1991). A gene encoding an antigen recognized by cytolytic T lymphocytes on a human melanoma. Science, 254(5038), 1643–1647. https://doi.org/10.1126/science.1840703.
- Coulie, P. G., Karanikas, V., Colau, D., Lurquin, C., Landry, C., Marchand, M., Dorval, T., Brichard, V., & Boon, T. (2001). A monoclonal cytolytic T-lymphocyte response observed in a melanoma patient vaccinated with a tumor-specific antigenic peptide encoded by gene MAGE-3. Proceedings of the National Academy of Sciences, 98(18), 10290–10295. https://doi.org/10.1073/pnas.161260098.
- Rosenberg, S. A., Yang, J. C., Schwartzentruber, D. J., Hwu, P., Marincola, F. M., Topalian, S. L., Restifo, N. P., Dudley, M. E., Schwarz, S. L., Spiess, P. J., Parkhurst, M. R., Kawakami, Y., Seipp, C. A., Einhorn, J. H., & White, D. E. (1998). Immunologic and therapeutic evaluation of a synthetic peptide vaccine for the treatment of patients with metastatic melanoma. Nature Medicine, 4(3), 321–327. https://doi.org/10.1038/nm0398-321.
- Chow, S., Berek, J. S., & Dorigo, O. (2020). Development of therapeutic vaccines for ovarian cancer. Vaccines, 8(4), 657. https://doi.org/10.3390/vaccines8040657.
- Rosenberg, S. A., Yang, J. C., & Restifo, N. P. (2004). Cancer immunotherapy: Moving beyond current vaccines. Nature Medicine, 10(9), 909–915. https://doi.org/10.1038/nm1100.
- Sahin, U., Derhovanessian, E., Miller, M., Kloke, B.-P., Simon, P., Löwer, M., Bukur, V., Tadmor, A. D., Luxemburger, U., Schrörs, B., Omokoko, T., Vormehr, M., Albrecht, C., Paruzynski, A., Kuhn, A. N., Buck, J., Heesch, S., Schreeb, K. H., Müller, F., … Türeci, Ö. (2017). Personalized RNA mutanome vaccines mobilize poly-specific therapeutic immunity against cancer. Nature, 547(7662), 222–226. https://doi.org/10.1038/nature23003.
- Wagner, S., Mullins, C. S., & Linnebacher, M. (2018). Colorectal cancer vaccines: Tumor-associated antigens vs neoantigens. World journal of gastroenterology, 24(48), 5418–5432. https://doi.org/10.3748/wjg.v24.i48.5418.
- Sacdalan, D. B., & Lucero, J. A. (2021). The Association Between Inflammation and Immunosuppression: Implications for ICI Biomarker Development. OncoTargets and therapy, 14, 2053–2064. https://doi.org/ 10.2147/OTT.S278089.
9. Cuzzubbo, S., Mangsbo, S., Nagarajan, D., Habra, K., Pockley, A. G., & McArdle, S. (2021). Cancer Vaccines: Adjuvant Potency, Importance of Age, Lifestyle, and Treatments. Frontiers in immunology, 11, 615240. https://doi.org/10.3389/fimmu.2020.615240. - Miao, L., Zhang, Y., & Huang, L. (2021). mRNA vaccine for cancer immunotherapy. Molecular cancer, 20(1), 41. https://doi.org/ 10.1186/s12943-021-01335-5.
- Sellars, M. C., Wu, C. J., & Fritsch, E. F. (2022). Cancer vaccines: Building a bridge over troubled waters. Cell, 185(15), 2770–2788. https://doi.org/10.1016/j.cell.2022.06.035.
- Cuzzubbo, S., Mangsbo, S., Nagarajan, D., Habra, K., Pockley, A. G., & McArdle, S. (2021). Cancer Vaccines: Adjuvant Potency, Importance of Age, Lifestyle, and Treatments. Frontiers in immunology, 11, 615240. https://doi.org/ 10.3389/fimmu.2020.615240.
- Hailemichael, Y., Dai, Z., Jaffarzad, N., Ye, Y., Medina, M. A., Huang, X. F., Dorta-Estremera, S. M., Greeley, N. R., Nitti, G., Peng, W., Liu, C., Lou, Y., Wang, Z., Ma, W., Rabinovich, B., Sowell, R. T., Schluns, K. S., Davis, R. E., Hwu, P., & Overwijk, W. W. (2013). Persistent antigen at vaccination sites induces tumor-specific CD8⁺ T cell sequestration, dysfunction and deletion. Nature medicine, 19(4), 465–472. https://doi.org/10.1038/nm.3105.
- Saxena, M., van der Burg, S. H., Melief, C., & Bhardwaj, N. (2021). Therapeutic cancer vaccines. Nature reviews. Cancer, 21(6), 360–378. https://doi.org/10.1038/s41568-021-00346-0.
- Bramhecha, Y., Dorigo, O., Kaliaperumal, V., Torrey, H., Ebrahimizadeh, W., Patterson, K., Dirk, B., Bydoun, M., Kennedy, B., Lobo, A., Weir, G., Graff, J., Fiset, S., & Hrytsenko, O. (2021). Abstract LBA026: Survivin peptides formulated in the DPX delivery platform rather than standard emulsions, elicit a robust, sustained T cell response to survivin in advanced and recurrent ovarian cancer patients. Poster Presentations – Late-Breaking Proffered Abstracts. https://doi.org/10.1158/1535-7163.targ-21-lba026.
- Ovarian cancer recurrence and treatment. OCRA. (n.d.). Retrieved May 19, 2022, from https://ocrahope.org/patients/about-ovarian-cancer/recurrence/.
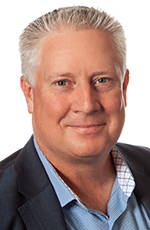
Dr. Jeremy Graff is the Chief Scientific Officer of IMV Inc. and brings more than 20 years of experience in preclinical and clinical research and translational analysis for novel immune-activating therapeutics in oncology. Most recently, Dr. Graff served as Chief Development Officer and Senior Vice President, Research at HiberCell, a biotechnology company developing novel therapeutics for cancer relapse and metastasis. He led the scientific and clinical development teams for HiberCell. Prior to that he was employed at Biothera Pharmaceuticals serving as President since 2018 and Chief Scientific Officer since 2014. In these executive roles, he implemented strategic translational studies along with clinical programs in immuno-oncology. He also managed corporate strategy for investor engagement and oversaw the acquisition of Biothera’s lead asset Imprime PGG by HiberCell, Inc in 2020. Dr. Graff spent 16 years at Eli Lilly and Lilly Research Labs, where he developed extensive experience in cancer drug discovery and development, immuno-oncology, biomarker discovery, and patient stratification. During his last position at Eli Lilly as Group Leader, Cancer Biology and Patient Tailoring, he established a Translational Oncology Unit to improve the technical success of clinical trials. At Lilly Research Labs, he was the recipient of President’s Recognition Award, the company’s highest annual award. Dr. Graff earned his PhD from the University of Kentucky’s Markey Cancer Center and completed a post-doctoral fellowship at the John Hopkins University Oncology Center. He has authored 60 peer-reviewed publications and holds a number of patents for novel cancer therapies.
Total Page Views: 6682