Issue:March 2024
PBPK MODELING - Critical Parameters for Simulating Oral Absorption Using PBPK Models
INTRODUCTION
Physiologically based pharmacokinetic (PBPK) modeling, along with its subset, physiologically based pharmaceutics modeling (PBBM), is a powerful tool for predicting drug behavior in vivo, including exposure and interactions with other drugs. Computer models are combined with in vitro assays to generate predictions to inform drug development activities, speeding development, and avoiding potential problems before they arise.
Identifying formulations to mitigate absorption risks is one application. Many drug candidates have characteristics, such as poor solubility or permeability, that jeopardize achieving target oral exposure. Using PBPK software to identify absorption risks requires knowledge of key drug formulation properties, the physiological properties of the gastrointestinal (GI) tract, and how the two interact. What are the properties, how can they be calculated or measured in vitro, and then used in PBPK simulations of in vivo performance?
SOLUBILITY
Dissolved drug concentration is a fundamental driver of drug bioperformance.1 It is determined by the solubility or by the activity of a metastable drug form. It drives the rate and extent of dissolution and precipitation in GI fluids, and the permeation rate across the GI membrane. Dissolved drug concentration is influenced by the interplay among the properties of the drug, excipients, and GI fluid. Key physiological parameters impacting dissolved drug concentration include GI fluid pH and composition, while key drug and excipient properties include solid form type, pH, lipophilicity, and melting temperature.2
Representing solubility in PBPK software is not as simple as entering a single number. Regional differences in GI fluid properties along the length of the GI tract make the situation dynamic, as does the potential for solid form conversions when a metastable solid form is present. Regional differences in solubility can be explained by differences in fluid pH between GI tract compartments, and in concentrations of mixed lipidic aggregates, such as bile salt micelles.
Fluid pH can alter the degree of drug ionization of weakly acidic and basic drugs. For example, a weakly basic drug is typically highly ionized at the acidic pH of the fasted human stomach, but mostly non-ionized at the moderate pH of the small intestine, giving higher solubility in the stomach than the intestine. This pH dependence makes it important to measure the pH-solubility profile of a drug as an input to allow simulation of the degree of ionization and therefore solubility in different GI tract compartments.
Drug solubilization by bile salt micelles present in some GI fluids makes it important to estimate solubility in the presence of bile through in vitro measurements of solubility in GI fluid surrogates, such as Fasted Simulated Intestinal Fluid (FaSSIF), which contains the solubilizing agents sodium taurocholate and lecithin. Measuring drug solubility as a function of FaSSIF concentration allows determination of a bile salt micelle partition coefficient, allowing PBPK software to calculate drug solubilization levels in each GI compartment (Figure 1).
As metastable solid forms can convert, it is important to include the solubilities of each relevant form in PBPK software. For example, in modeling an amorphous solid dispersion, the pH-solubility profile and solubilization ratio of both the amorphous form, as well as of any crystalline forms to which the drug is likely to precipitate in vivo, are required inputs to account for the lower activity and dissolution rate of the latter forms.
DISSOLUTION
Solid oral dosage forms must dissolve in GI fluids before they can absorb across the GI membrane and enter the bloodstream. The rate and extent of dissolution is thus an important input.1 Like solubility, the interplay between drug substance, excipient, and physiological properties influences in vivo drug dissolution.3 Solubility is a key driving force for dissolution, together with other key factors such as the solid surface area, concentration of drug in solution, and fluid hydrodynamics. To improve reliability when mathematically inputting dissolution into PBPK software, one must consider these important variables.
In vitro dissolution testing is the gold standard for forecasting in vivo drug product bioperformance. It can be used to generate inputs to PBPK software, such as the rate and extent of dissolution and precipitation as a function of properties, such as pH and bile salt concentrations. It is helpful to tailor the methodology to the target population/physiology and drug product problem statement by using a variety of customized, fit-for-purpose dissolution apparatuses.4
Some examples of simple in vitro dissolution apparatuses include the small-scale Pion μDiss Profiler™ for dry powders and suspensions, and the larger-scale United States Pharmacopeia (USP) 1 and 2 apparatuses for dosage forms, including tablets and capsules. These tests can be run using a single dissolution medium targeting dissolution in a particular region of the GI tract. Alternatively, they can be run as transfer-tests, in which the drug formulation is added to a simulated gastric medium, and a concentrated simulated intestinal buffer is subsequently added to create a simulated intestinal medium.4
For either single medium or transfer in vitro dissolution tests, the rate and extent of dissolution are monitored by measuring the dissolved drug versus time and incorporated into PBPK simulations. Ideally, a mechanistic or semi-mechanistic dissolution equation is selected to translate in vitro dissolution to predictions of in vivo dissolution. Such equations calculate dissolution as a function of fluid properties (eg, pH, concentration of bile salts), dose, and dissolved drug concentration, properties that may differ between the in vitro test(s) and simulation parameters. Examples of semi-mechanistic dissolution equations include the z-factor, Wang-Flanagan, Johnson, and product particle size (P-PSD) models (Figure 2).5
PRECIPITATION
Drug molecules can precipitate in gastrointestinal fluids to form solid drug particles when they are dissolved at concentrations exceeding their thermodynamic solubility. Precipitation undermines absorption as it can reduce the driving force for permeation across the GI membrane. Supersaturated concentrations can occur when dissolved drug is introduced into the GI tract above its thermodynamic solubility in that region. For example, a weakly basic drug with high solubility in an acidic stomach may transit to the small intestine at a supersaturated concentration.6 Further, a drug formulated as an amorphous solid dispersion, salt form, or metastable polymorph might dissolve to the solubility of that enabled form, which is supersaturated with respect to the solubility of the thermodynamically stable form. In all these cases, precipitation can occur.
Not all drugs precipitate in vivo, even under supersaturated conditions, and this depends upon factors such as solubility, degree of supersaturation, drug properties, GI fluid composition, and formulation components.6 In vitro methods are often used to determine the tendency of a drug to precipitate from a formulation and quantify the rate of precipitation for input into PBPK software.
A useful in vitro method for estimating precipitation rate is the transfer test previously described. In vitro transfer tests should incorporate physiological GI fluid properties and composition, and a relevant drug dose. For example, the relevant in vitro dose concentration might be selected by dividing the in vivo dose by the estimated GI fluid volume. Ideally, multiple concentrations would be tested to explore the impact of dose-to-volume on degree of supersaturation and the potential for precipitation. Alternatively, there are more sophisticated in vitro tests that allow for more physiologically relevant supersaturation ratios, perhaps by including fluid transit and/or an absorption compartment, that can provide greater insight into precipitation.7
Drug precipitation rate can be tracked by monitoring the decline in drug concentration versus time. Precipitation rate can be translated to predictions of in vivo precipitation by, for example, a first-order fit to the data to determine a mean precipitation time (MPT).5 MPT can be input into PBPK software, allowing drug precipitation to be simulated when drug concentration exceeds the solubility. Multiple values for MPT can be defined to account for differences in precipitation rate for different GI compartment or pH values (Figure 3).
PERMEATION
To access the bloodstream, drug permeates from GI fluids across the GI membrane by passive diffusion and/or multiple active transport processes. Key parameters impacting the rate and extent of permeation include regional effective permeability across the intestinal wall, surface area available for absorption, and drug species type and concentration at the intestinal wall.8 These parameters depend on formulated drug properties, including lipophilicity, size, and charge, and GI fluid properties such as intestinal wall composition and fluid hydrodynamics.
Human effective permeability (Peff) is a key input to PBPK software used to simulate in vivo permeation. Peff can be predicted using quantitative structure-activity relationship models, measured using in vitro models of permeability, such as through human intestinal epithelial cells, originated from Madin-Darby canine kidney (MDCK) cells or human Caco-2 cells, or using in situ animal models.8 No single method is a reliable surrogate for in vivo Peff, but they are particularly useful when reference compounds with known Peff are used.
When inputting effective permeability derived from cell monolayers or in situ animal models, PBPK software can use built-in correlations to convert these data into Peff in humans or in animal species such as rat or dog. To simulate the rate of drug permeation, PBPK software uses Peff in addition to variables accounting for the effective permeation surface area in a given GI compartment, fluid flow rate, and drug concentration.
PUTTING PBPK VARIABLES INTO CONTEXT
Solubility, dissolution, precipitation, and permeation are integral to predicting the rate and extent of oral drug absorption. Their relative importance depends upon the drug, formulation, and physiological parameters, such as species and prandial state. Determining input parameter importance, or sensitivity, can be done within PBPK software by conducting parameter sensitivity analyses (PSAs) to determine which parameters significantly impact simulation outputs (Figure 4).
Careful attention should be paid to highly sensitive parameters, as small differences in their values cause large differences in simulation outputs. When sensitive parameters are identified, one should evaluate the robustness of that input and determine if more in vitro and/or in vivo experimentation is needed to gain confidence in the input value.
With an increasing number of drugs posing absorption challenges, the ability to predict how a new molecule will behave in vivo is important. Being able to predict and mitigate absorption problems before they arise should help the project progress with fewer delays.
PBPK is a useful tool for predicting absorption challenges and guiding formulation selection early in development when used with in vitro test methods tailored to the drug, formulation, and product properties. As more in vitro and in vivo data are gathered throughout the drug development process, early PBPK predictions can be refined and validated. This “learn and confirm” process facilitates informed decision-making throughout the drug lifecycle, aiding important drug development activities, such as setting clinically relevant specifications, developing biopredictive dissolution methods, food effect evaluation, and pH-dependent drug-drug interaction assessment.9,10
REFERENCES
- Amidon GL, Lennernäs H, Shah VP, Crison JR. A theoretical basis for a biopharmaceutic drug classification: the correlation of in vitro drug product dissolution and in vivo bioavailability. Pharm Res. 1995 Mar;12(3):413-20. doi: 10.1023/a:1016212804288. PMID: 7617530.
- Fagerberg JH, Bergström CA. Intestinal solubility and absorption of poorly water soluble compounds: predictions, challenges and solutions. Ther Deliv. 2015;6(8):935-59. doi: 10.4155/tde.15.45. Epub 2015 Aug 28. Erratum in: Ther Deliv. 2016 Jun;7(6):412-6. PMID: 26316058.
- Mudie DM, Samiei N, Marshall DJ, Amidon GE, Bergström CAS. Selection of In Vivo Predictive Dissolution Media Using Drug Substance and Physiological Properties. AAPS J. 2020 Jan 27;22(2):34. doi: 10.1208/s12248-020-0417-8. PMID: 31989343; PMCID: PMC6985051.
- Mudie DM, Selecting In Vitro Dissolution Methodologies For Amorphous Solid Dispersions. Lonza-White-Paper.pdf (d2akihtr51eb46.cloudfront.net).
- Gray, Vivian A., et al. “The case for physiologically based biopharmaceutics modelling (PBBM): what do dissolution scientists need to know.” development 12 (2020): 14.
- Thakral NK, Meister E, Jankovsky C, Li L, Schwabe R, Luo L, Chen S. Prediction of in vivo supersaturation and precipitation of poorly water-soluble drugs: Achievements and aspirations. Int J Pharm. 2021 May 1;600:120505. doi: 10.1016/j.ijpharm.2021.120505. Epub 2021 Mar 19. PMID: 33753162.
- Patel S, Zhu W, Xia B, Sharma N, Hermans A, Ehrick JD, Kesisoglou F, Pennington J. Integration of Precipitation Kinetics From an In Vitro, Multicompartment Transfer System and Mechanistic Oral Absorption Modeling for Pharmacokinetic Prediction of Weakly Basic Drugs. J Pharm Sci. 2019 Jan;108(1):574-583. doi: 10.1016/j.xphs.2018.10.051. Epub 2018 Nov 3. PMID: 30395833.
- Dahlgren D, Lennernäs H. Intestinal Permeability and Drug Absorption: Predictive Experimental, Computational and In Vivo Approaches. Pharmaceutics. 2019 Aug 13;11(8):411. doi: 10.3390/pharmaceutics11080411. PMID: 31412551; PMCID: PMC6723276.
- Food and Drug Administration. The Use of Physiologically Based Pharmacokinetic Analyses Biopharmaceutics Applications for Oral Drug Product Development, Manufacturing Changes, and Controls. https://www.fda.gov/media/142500/download.
- European Medicines Agency, Guideline on the reporting of physiologically based pharmacokinetic (PBPK) modelling and simulation. 2020. Guideline on the reporting of physiologically based pharmacokinetic (PBPK) modelling and simulation. https://www.ema.europa.eu/en/documents/scientific-guideline/guideline-reporting-physiologically-based-pharmacokinetic-pbpk-modelling-simulation_en.pdf.
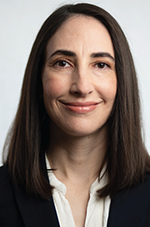
Dr. Deanna Mudie is a Senior Principal Engineer in Research and Development at Lonza’s site in Bend, OR. She earned her BSE in Chemical Engineering and her PhD in Pharmaceutical Sciences from the University of Michigan. Since joining Lonza in 2016, she has focused on enabling bioavailability-enhancing amorphous solid dispersion (ASD) formulations by developing dosage form platforms and in vitro/in silico strategies for predicting ASD bioperformance. During her doctoral and post-doctoral work, she developed mechanistic in vitro and in vivo drug transport models to predict oral dosage form dissolution and intestinal absorption. She began her career in the pharmaceutical field as an engineer at Pfizer and Merck characterizing, developing, and manufacturing oral dosage forms from preclinical to commercial scales.
Total Page Views: 3966