Issue:January/February 2025
OXYGEN SCAVENGING - Innovative Oxygen Scavenging Technologies for Pharmaceutical Packaging: Ensuring Drug Integrity & Stability
ABSTRACT
Oxygen scavenging is a vital strategy in pharmaceutical packaging, aimed at preventing the oxidation and degradation of drugs. By incorporating novel active materials science technology into active packaging solutions to actively remove oxygen from the packaging headspace, pharma companies can ensure the stability and efficacy of products throughout shelf-life. Sophisticated oxygen scavenging technology can be seamlessly integrated into existing packaging formats and processes as pieces of film (heat-staked films, blown films, etc), minimizing disruption to manufacturing workflows.
INTRODUCTION
Oxygen scavenging in food and pharmaceutical packaging is a critical technology designed to enhance the stability and shelf-life of various drug and food products.1,2 Oxygen, even in trace amounts, can lead to the oxidation and subsequent degradation of active pharmaceutical ingredients (APIs), impacting their efficacy, safety, and appearance. The oxidation process can cause the formation of harmful by-products, reduce the potency of APIs, and alter physical characteristics, such as color and solubility. Consequently, controlling and minimizing oxygen levels within pharmaceutical packaging is paramount to maintaining drug quality. The application of oxygen scavenging technology is especially important for those pharmaceuticals particularly sensitive to oxidation, such as certain antibiotics, vitamins, and biologics. Notably, the use of oxygen scavengers can reduce the need for preservatives and antioxidants within the formulation, potentially lowering risk of adverse reactions.
Varying strategies can be used to address risks initiated by the presence of oxygen inside the packaging headspace.3 Common methods used to mitigate oxygen in packaging include the following:
Barrier Materials: Packaging with high oxygen barrier properties can be used to prevent external oxygen from entering the package.4
Modified Atmosphere Packaging (MAP): In MAP, the atmosphere inside the package is adjusted by replacing oxygen with inert gases (eg, nitrogen, carbon dioxide).5
Chemical Scavengers: These include organic scavengers (ascorbic acid, ascorbic acid salts, isoascorbic acid, tocopherol, hydroquinone, catechol, rongalite, sorbose, lignin, gallic acid, lecithin, rosemary extracts, polyunsaturated fatty acids, threonine), metallic scavengers (iron powder, activated iron, ferrous oxide, iron salt, iron nanoparticles, Co (II), Zn, platinum, palladium), inorganic (non-metallic) scavengers (sulfite, thiosulfate, dithionite, hydrogen sulfite, titanium dioxide) and polymer based scavengers (oxidation-reduction resin, polymer metallic complex).6-8
Enzymatic Scavengers: The most prominent are glucose oxidase, laccase, and ethanol oxidase. Enzymes like glucose oxidase catalyze the conversion of glucose to gluconic acid and hydrogen peroxide. The latter reacts with oxygen, reducing its levels.9
The first two strategies (barrier materials and modified atmosphere packaging) can be utilized to protect unit dose (individual) drug packaging, but are inefficient for packaging containing several doses because external oxygen can enter the packaging as the user repeatedly opens and closes it. One widely used solution is oxygen scavenging materials, which can be incorporated into packaging systems to protect sensitive products by actively removing oxygen from the surrounding environment. These scavengers can be integrated into various components of the packaging, such as bottle closures, blister packs, or sachets, and operate through chemical reactions that absorb or adsorb oxygen. Commonly used oxygen scavenging materials include iron powder and ascorbic acid, either of which is chosen based on the specific requirements of the drug product and packaging design.
Iron-based scavengers work through a process of oxidation where iron reacts with oxygen to form iron oxide, effectively reducing oxygen concentration.6 Ascorbic acid, another widely used scavenger, reacts with oxygen to form dehydroascorbic acid. Non-metallic scavengers can also be a solution when metallic systems are not permitted. Among these, sulfites are highly effective oxidation compounds.10
An alternate technology, not requiring water, that produces peroxyl radicals and ultimately acts as an oxygen scavenger has also been of interest. In this technology, peroxyl radicals arise from the direct interaction between oxygen and alkyl radicals. The formation of peroxyl radicals are limited to the oxygen available in the headspace in a sealed package. An unconjugated chain reacts with the peroxyl radicals to capture the oxygen. The bonds then rearrange to form an oxygen-bound conjugated chain. Figure 1 depicts this reaction. Depending on the length of the chain, multiple oxygen molecules could be captured. Once the oxygen is bound to the hydrocarbon chain, the compound is very stable. These types of scavengers can be modified with photo initiators to prevent premature oxidation of the scavenger during processing. Enzyme-based oxidation is another approach to regulating the oxygen concentration in food packages. Enzymatic scavengers employ enzymes to catalyze reactions that convert oxygen into less reactive species. These materials are often selected based on their compatibility with food and drug products, the desired rate of oxygen removal, and regulatory considerations. Some of them can start scavenging oxygen immediately after exposure to oxygen or air at ambient humidity and temperature. On the other hand, some oxygen scavengers require an activation mechanism (water vapor, ultra-violet light or magnetic fields, for example), that helps control the initiation of the reaction, allowing the oxygen scavenging system to be stable before use with drugs and avoiding its premature activation.
The following explores how chemical oxygen scavengers can be deployed using a novel active polymer platform to scavenge oxygen. Such an approach can substantially limit the amount of oxygen in the packaging headspace that could otherwise cause deteriorative reactions and, ultimately, lead to reduced drug functionality.
METHODS
Pioneered by Aptar CSP Technologies, the 3-Phase Activ-Polymer™ platform technology employs active materials as fillers in composite materials (Figure 2). The proprietary technology is delivered in a unique formulation comprised of a base majority polymer that provides the structure, a channeling agent, and active particles. In this case, the polymer was deployed as an active film.
As a first step, five different raw active chemical oxygen scavengers (three using humidity as a trigger to scavenge oxygen, and two that work in dry conditions) and one enzymatic scavenger were engineered for inclusion into a polymer matrix (Figure 3). The incorporation of oxygen scavenging systems into the polymer matrix may affect the functional properties of the plastic, including the tensile strength, elongation, gas barrier, thermal properties, and optics. Therefore, the chemical and physical compatibility with the scavenger, as well as the stability with the active phase during the manufacturing processes, were considered when developing the oxygen scavenging films. A good dispersion of the scavenging agent by sufficient shear during the extrusion process was also achieved, as shown from the homogeneity of the dispersion of the active materials in the polymer matrix of oxygen scavenging film #2 (Figure 2).
Twelve glass bottles (V = 120 mL) were cleaned and prepared with oxydot, and a 1 x 1 in2 piece of one of six different active films was placed in the bottle (two bottles per each type of film) (Figure 3). For the wet oxygen scavenging system, a 1 x 1 in2 filter paper impregnated with a certain volume of water (Table 1) was also added to the bottle.
The bottles were then crimp sealed and analyzed by the OxySense system (Gen III, 5000 series) for an initial oxygen reading before being stored in a 25⁰C/ 60% RH environmental chamber. Using the OxySense system, two samples of each type of the six films (Figure 3) were tested daily for 200 days.
OxySense uses a luminescence method to calculate the amount of oxygen in a closed system. Oxygen absorption rate (OAR) tests were executed for both the wet and dry oxygen scavenging films (Table 1). Once the OAR data was collected, the percent change of oxygen in each bottle was calculated by subtracting the final percentage of oxygen in the bottle from the initial percentage. The amount of oxygen present in the bottle was calculated by multiplying the volume of the bottle (120 mL) by the percentage change of oxygen. Then, the OAR was calculated by dividing the amount of oxygen present by elapsed time.
RESULTS & DISCUSSION
Figure 4 shows the OAR for the other six oxygen scavenging films for the full 200 days.
O2 Film #5 and O2 Film #6, which can react with oxygen in the absence of humidity, show the highest OAR value among the films that can be used for 200 days. O2 Film #5 displayed an OAR of 0.060 cc/day. O2 Film #6 displayed the highest OAR value of 0.073 cc/day, 0.013 cc/day higher than O2 Film #5.
The capacity of the films was then calculated after the full data set was collected. First, the amount of oxygen left in the bottle was calculated by multiplying 120 mL by the minimum oxygen reading in the data set. Next, the amount of oxygen scavenged was determined by subtracting the oxygen left in the bottle from the original amount of oxygen in the bottle, which was assumed to be 21% oxygen. Then, the capacity was calculated by dividing the amount of oxygen scavenged by the surface area of the sample, which was 1 in2 or 6.45 cm2. Figure 5 below shows the capacities of the films tested.
The capacity calculated for O2 Film #5 is 2.19 cm3/cm2, which is higher than most of the other films tested. The capacity calculated for O2 Film #6 was the highest (2.60 cm3/cm2), which is 16% higher than O2 Film #5.
CONCLUSION
Oxygen scavengers will continue to play a crucial role in pharmaceutical packaging by protecting drugs from oxidative degradation. As manufacturers seek to optimize costs and maintain drug potency, these scavengers help extend shelf life and prevent adverse effects caused by oxygen exposure. Incorporating scavengers directly into packaging can help pharma developers mitigate degradation risk, enhance stability, and meet regulatory requirements without the need to reformulate their drugs.
Aptar CSP Technologies’ novel 3-Phase Activ-Polymer™ oxygen scavenging films, which actively remove oxygen from packaging headspace, can significantly extend the shelf life of drugs subjected to oxidative degradation, ensuring they remain effective until the end of their intended use.
REFERENCES
- Apicella, A., Incarnato, L., Oxygen Scavengers in Food Packaging, Reference Module in Food Science, Elsevier, 2019, ISBN 9780081005965.
- Dey, A., Neogi, S., Oxygen scavengers for food packaging applications: A review. Trends in Food Science & Technology, 2019, 90, 26-4.
- Gaikwad, K.K., Singh, S., Lee, Y.S., 2018. Oxygen scavenging films in food packaging. Environ. Chem. Lett., 16, 523–538.
- Zabihzadeh Khajavi, M., Ebrahimi, A., Yousefi, M. et al., Strategies for Producing Improved Oxygen Barrier Materials Appropriate for the Food Packaging Sector. Food Eng. Rev., 2020, 12, 346-363.
- Han, J.H., A review of food packaging technologies and innovations, Innovations in food packaging (second edition). Academic Press, 2014, 3-12.
- Johansson, K., Jönsson, L., Järnström, L., 2011. Oxygen scavenging enzymes in coatings – effect of coating procedures on enzyme activity. Nord. Pulp Pap. Res. J., 26, 197-204
- Miltz, J., Perry, M., Evaluation of the performance of iron-based oxygen scavengers, with comments on their optimal applications. Packag. Technol. Sci., 2005, 18, 21-27.
- Damaj, Z., Joly, C., Guillon, E., 2015. Toward new polymeric oxygen scavenging systems: formation of poly(vinyl alcohol) oxygen scavenger film. Packag. Technol. Sci., 28, 293-302.
- Scarfato, P., Avallone, E., Galdi, M.R., Di Maio, L., Incarnato, L., Preparation, characterization, and oxygen scavenging capacity of biodegradable a-tocopherol/PLA microparticles for active food packaging applications. Polym. Compos., 2015, 38, 981-986.
- Brody, A.L., Strupinsky, E.P., Kline, L.R., Active Food Packaging for Food Applications. CRC Press, Boca Raton, FL, 2001.
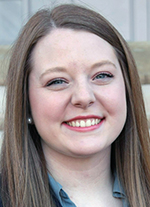
Amanda Murph is a Scientist II in the Research and Applications Development division at Aptar CSP Technologies. With a Bachelor’s degree in Materials Engineering, she demonstrates a strong commitment to innovation and collaborative problem-solving by consistently exploring novel material applications and focusing on achieving tangible results.
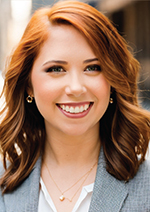
Ivy Comer, a Scientist III in the Applications and Research Development Team at Aptar CSP Technologies, holds a Bachelor of Science in Biochemistry from Auburn University. Her expertise in innovation, analytical methodologies, and strategic problem-solving has driven impactful solutions in the medical and packaging industries.
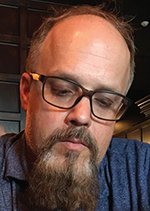
Jason D. Pratt is Director of Material Science at Aptar CSP Technologies with a passion for bringing innovative solutions to commercial opportunities. Skilled at bringing together and mentoring high power teams to tackle impossible problems, he is a green chemist with over 40 patents across the food, cosmetics, pharma and construction industries.
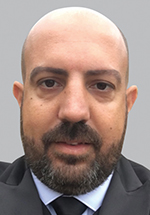
Dr. Jean Daou, after earning a PhD in Material Chemistry from the University of Louis Pasteur, joined the University of Haute Alsace as Assistant Professor and was promoted to full professor in 2016. In 2021, he joined Aptar CSP Technologies as R&D Manager, where his research activities focus on the synthesis of porous materials, the study of their properties and their shaping to meet pharmaceutical packaging demands for molecular decontamination. He is the author of 137 publications in international journals and 18 patents.
Total Page Views: 2557