Issue:March 2021
ORAL MUCOSAL IMMUNOTHERAPY - Oral Mucosal Delivery of Allergenic Proteins for Inducing Tolerance in Food Allergic Individuals
INTRODUCTION
Currently, there is a tremendous amount of interest in the delivery of drugs through the oral mucosa for a vast array of medical conditions. The oral mucosa is more permeable than the skin by 1000-fold and lacks the enzymatic activity of the gastrointestinal tract.1 One of the key benefits of this route, however, is the absence of first-pass metabolism. When a drug is delivered directly into the systemic circulation, rather than allowing it to absorb through the stomach and intestinal lining, it bypasses the hepatic venous system and avoids being metabolized by the liver. This can enhance the efficacy of a drug, theoretically lowering the therapeutic dose required. Other routes of drug delivery outside the gastrointestinal tract, such as injection and transdermal application, may be associated with increased discomfort, inconvenience, or undesirable side effects. Oral mucosal delivery is also very useful for children or adults who have difficulty swallowing tablets, or for people with gastrointestinal illnesses that are experiencing nausea, vomiting, or dysphagia.2
Pharmacokinetic data has been collected for the oral mucosal delivery of hundreds of small molecule drugs, commonly using the buccal or sublingual surfaces, of which the goal is for the drug to pass into the circulation. In contrast, the goal of oral mucosal delivery for allergy immunotherapy is to concentrate the large extract proteins in the mucosa itself, rather than promoting entry into the circulation, so that they can interact with the abundant levels of antigen presenting cells (oral Langerhans cells, or oLC) in this area, leading to immune tolerance.3 The oral mucosal delivery of allergenic polypeptides for the purpose of allergy immunotherapy, or desensitization, has been in routine clinical use for several decades to treat respiratory allergies.
Oral mucosal delivery of allergenic proteins for respiratory allergies has been found to alleviate symptoms and reduce medication use, on a level comparable with subcutaneous immunotherapy (SCIT, or allergy shots); this method is also associated with improvements in quality of life, prevention of asthma, and a superior safety profile.4,5 Oral mucosal immunotherapy (OMIT) for respiratory allergies has been commonly delivered through the sublingual mucosa, using either liquid extract drops or fast-dissolving tablets (also referred to as sublingual immunotherapy, or SLIT). More recently, a specialized toothpaste delivery vehicle (Allerdent®, Allovate, LLC) has been developed to stabilize the allergenic extract proteins and release them upon regular tooth brushing to a wider area of buccal, vestibular, lingual, and sublingual mucosal surfaces.6 Further, INT301 (Intrommune Therapeutics), is an OMIT product being developed for the treatment of peanut allergies. INT301’s unique formulation is designed to desensitize an individual with peanut allergy using a toothpaste delivery system, protecting them in the event of accidental peanut exposure. This innovative OMIT toothpaste delivery system has been shown to enhance adherence to therapy because it is linked to a common daily activity, and exhibits fewer gastrointestinal side effects because it is not swallowed.
In fact, OMIT is safe enough to be performed as a home therapy. This has led to new strategies for desensitization against food allergies, a treatment proven to be too dangerous for clinical use when delivered by subcutaneous injection.7 Kim and colleagues demonstrated long-term clinical and immunologic tolerance, along with a low rate of adverse events, when children were treated with liquid peanut extract applied to the sublingual space over a period of 3 to 5 years.8 Oral immunotherapy (OIT), using swallowed peanut protein flour, has also been found to produce a robust degree of desensitization, but is associated with a higher degree of serious adverse events, including anaphylaxis.9 This paper will highlight some of the differences between food allergy immunotherapy via the oral mucosal route versus exposure through the stomach and intestines.
THE PHARMACOKINETICS OF ORAL MUCOSAL DELIVERY
Saturation Solubility
The journey for allergenic proteins entering the oral cavity and encountering the mucosa begins with the saturation solubility, or how much of the protein gets dissolved in the liquid/slurry from the protein application modality plus the roughly 1 ml of saliva that is present in the oral cavity.10 If the protein level is above saturation, the absorption into the mucosa requires a longer exposure, because some would have to first be absorbed into the mucosa, allowing the excess protein in the mouth to then dissolve in the saliva. In one study using SLIT, 26% to 30% of the allergenic protein was expelled in the saliva, presumably because the small surface area of the sublingual space kept saturation levels high.11 The interaction of the protein molecule with the mucosal membrane is also facilitated by ionic forces, whereby the slightly positive charged protein molecules binds to the slightly negatively charged mucosal cells. In the case of OMIT using the toothpaste delivery vehicle, it is believed that brushing across the mucosal surface also removes some of the epithelial debris and keratin, exposing more of the area available for absorption.
Distribution Equilibrium
The second part of the process is the distribution equilibrium, which directs the actual absorption of the allergenic extract proteins into the mucosa. This is a rapid process, with an estimated mean absorption half-life of 52 seconds.10 Rapid clearance of the protein from the oral cavity, however, does not imply rapid systemic exposure. The mucosa acts as a reservoir, reaching an equilibrium with the protein in the saliva/foam in the mouth. This is independent of the dose of protein in the mouth. In other words, once the mucosa has fulfilled its quota in the region, more drug will stay in the mouth and be swallowed or expelled, as previously noted. This raises concern that concentrating the proteins in liquid sublingual drops could lead to a wasting of excess protein due to oversaturation in one area. Thus, distributing the protein to a wider area would be potentially very beneficial in terms of utilizing all available mucosal surfaces. Even after extensive mouth rinsing, 2% of the radiolabeled allergen remains in the oral cavity mucosa 20 hours after administration, suggesting that processing of the allergen was still occurring within the mucosal reservoir.10
Bioavailability
The bioavailability, or how much of a drug or protein reaches the systemic circulation, is largely independent of the dose delivered into the oral cavity because of the partitioning phenomenon of the oral mucosa. Drinking water 2 minutes after sublingual administration of Asenapine, an anti-psychotic drug, does not affect the speed of bioavailability.10 This would be analogous to a car, which can run at the same speed whether or not it has a half-filled tank or a full tank. Whether or not the drug will reach the circulation is based more on the characteristics of the molecule itself, such as size, molecular weight, configuration, how extensively it is processed, pH, affinity for blood vessels, ability to penetrate blood vessel endothelium, and interactions with other cells in the mucosa. Peanut proteins, which are well-known to cause anaphylaxis, are large molecules that are unable to cross the oral mucosal membrane without the assistance of oLCs. Ara h 2, one of the major peanut allergen proteins with a molecular weight of 17,000 g/mol, whether avidly bound to oLC or not, does not have a realistic pathway to reach the systemic circulation under normal conditions, particularly if blood is actively flowing out of a small vessel. Nonetheless, temporarily suspending OMIT treatment when open wounds, ulcers, lesions, or significant gum bleeding are present is a prudent recommendation.
In general, intact proteins are not transported directly into the bloodstream. The exceptions to this rule are the placenta, where immunoglobulins can be transferred directly to the fetal blood supply, and the human small intestine in the very first few weeks of life, where a “leaky” mucosa helps facilitate absorption of immunoglobulins present in breast milk.12 Normally, the cells of the GI tract absorb digested proteins and then transport the fragments, which are rarely more than four amino acids long, into the bloodstream. In contrast, Ara h 2 has over 100 amino acids and a complex 3D structure. The oLC do not process food proteins to a point when they are small enough to gain access to the circulation, and therefore preferentially transports them to locoregional lymph nodes for processing. It has also been suggested that ongoing gut leakiness, either through disease or medications, such as proton pump inhibitors, promotes an ongoing cycle of allergic inflammation that increases the risk of developing food allergies.13
MOUSE STUDIES
Immunoglobulin E (IgE) from C3H/HeJ mice can recognize the same peanut allergen isoforms and epitopes as IgE from human peanut-allergic patients, making it a good model for anaphylaxis.14 Mouse studies have also shown that dendritic cells from the oral mucosa acquire allergenic proteins in the buccal epithelium and transport them toward the draining lymph nodes, where they are processed and presented to MHC class II-restricted T cells.15 Specific IgE and IgG responses were actually decreased when dosing newborn Brown Norway rats in the oral cavity with ovalbumin, as opposed to the allergenic priming effect seen when the exposure was through intragastric intubation. This supports a distinct pathway of antigen presentation in the oral mucosa, as well as a robust protective effect from early exposure of allergens to these tissues.16 The distribution of a sublingually applied solution of Timothy grass allergen in sensitized mice has been investigated using 1% Evans Blue solution in phosphate-buffered saline (PBS) with 1.2% carboxymethylcellulose (CMC, an ingredient commonly seen in toothpaste, including Allerdent®, and other cosmetic products).17 Thirty minutes after sublingual administration, the dye was detectable in the sublingual tissue, but not in the esophagus or in the stomach. Without using CMC, the dye was also found in the stomach, demonstrating the positive impact of using viscous materials to support longer retention of proteins delivered to the oral cavity mucosa.
After Japanese Cedar pollen was painted on the sublingual and buccal mucosal surfaces in BALB/c mice, there was a rise in the oLC population at 2 hours, which peaked by 10 hours, after which the activated oLC population decreased and was gone by 24 hours in both groups.18 The density of oLC was found to be lower in the sublingual mucosa than in the buccal mucosa, consistent with the human studies performed by Allam and colleagues.3 With repeated paintings, equal percentages of resident and newly recruited oLC in the regional lymph nodes suggested that there was a consistent supply of new oLC at local buccal mucosa sites. In contrast, the supply of new oLC to the sublingual mucosa was very limited, demonstrating a marked decrease of oLC recruitment with repeated paintings and supporting the benefit of allergenic protein application to regions outside the sublingual space.
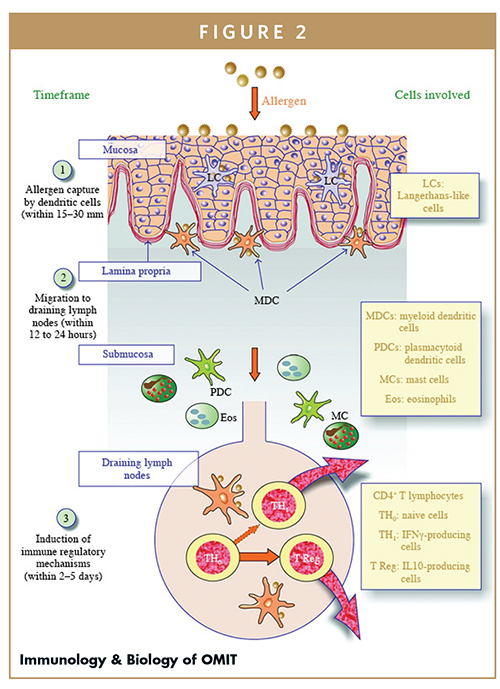
Adapted from Moingeon P, Mascarell L. Clin Dev Immunol. 2012;2012:623474.
HUMAN STUDIES
In healthy, non-allergic individuals given radiolabelled allergen by the sublingual route, no significant deiodination or degradation of the protein was noted, even after the allergen was kept under the tongue for 20 minutes.19 Serum detection of radioactive iodine alone and radioactive iodine-labeled small peptides increased only after swallowing, providing further evidence that large, intact peptides cannot gain access to the circulation via the oral mucosa under normal circumstances. In another study, plasma levels were achieved in sublingual-swallow patients only after swallowing and digestion within the stomach, with peak levels seen at 2 hours.11
Evidence suggests that even small molecules will have difficulty accessing the circulation through the oral cavity unless they are destined to do so by virtue of their inherent properties, such as 3D structure, pH, ionic charge, or biologic activity. Teeguarden and colleagues determined that oral exposure of bisphenol A, a monomer with a molecular weight of 228 g/mol, equivalent to the 95% upper percentile of total daily exposure, would produce less than 1% absorption through the mucosa.20 By using computer modeling of the oral cavity, compounds with a low rate of diffusion are predicted to have negligible systemic absorption, and that regions with a thicker mucosa, such as the buccal mucosa with 40-50 layers of non-keratinizing cells, would have a slower diffusion when compared with the relatively thin mucosa of the sublingual space.21
In the case of food allergy, both OMIT, applied to the sublingual space and/or other regions of the oral cavity, and OIT, whereby swallowed food proteins in powder reach the lymph tissue of the intestines, have been shown to be effective in promoting tolerance.8 One of the key differences between these methods is that OIT uses a much larger amount of allergenic food protein, inducing a robust clinical effect but also producing a higher risk of adverse allergic events compared to the oral mucosal route. Recent studies have also determined that the mechanism of action is different for these two routes of delivery. Each route stimulates a different subpopulation of dendritic cells, which are antigen-presenting cells that are essential for the development of tolerance, leading to different patterns of cytokine modulation.22 These findings also suggest that these two methods of food allergen delivery might be combined to function in a complementary fashion.23
SUMMARY
The accelerated interest in drug delivery via the oral mucosa, coupled with the significant increase in severe food allergies throughout the world, has shined a spotlight on oral mucosal immunotherapy. The World Allergy Organization (WAO) 2013 Position Paper on sublingual immunotherapy stated that “compared to the sublingual region, targeting the vestibule with allergen vaccine has the potential to induce enhanced immune deviation or tolerance, possibly with a lower potential for mast cell-related local side effects.”24 This position reflects the understanding that immunologically active, tolerance-producing cell populations are present in a high degree in regions beyond the sublingual space, a target traditionally used because of easy access and the pull of gravity. With more advanced delivery techniques, such as a buccal patch or a toothpaste vehicle, however, food allergy proteins can now reach these areas and gain access to the locoregional lymph nodes through an elegant escort system orchestrated by oral Langerhans cells. It is important to remember that one of the main functions of the oral cavity mucosa is to teach the immune system what is safe to consume.
With the recent FDA approval of the first oral immunotherapy platform for peanut allergy, improved platforms using oral mucosal immunotherapy are sure to follow for other food allergies, along with multi-food products.
REFERENCES
- Shojaei AH. Buccal mucosa as a route for systemic drug delivery: a review. J Pharma Sci. 1998;1:15-30.
- Giannola LI, Sutera FM, De Caro V. Physical methods to promote drug delivery on mucosal tissues of the oral cavity. Expert Opin Drug Deliv. 2013;10(10):1449-1462.
- Allam JP, Stojanovski G, Friedrichs N, et al. Distribution of Langerhans cells and mast cells within the human oral mucosa: new application sites of allergens in sublingual immunotherapy. Allergy. 2008;63:720-727.
- Wise SK, Schlosser RJ. Subcutaneous and sublingual immunotherapy for allergic rhinitis: what is the evidence? Am J Rhinol Allergy. 2012;26(1):18-22.
- Lin SY, Erekosima N, Kim JM, et al. Sublingual immunotherapy for the treatment of allergic rhinoconjunctivitis and asthma: a systematic review. JAMA. 2013;309 (12):1278-1288.
- Reisacher WR, Suurna MV, Rochlin K, Bremberg MG, Tropper G. Oral mucosal immunotherapy for allergic rhinitis: a pilot study. Allergy Rhinol. 2016;7:1-8; doi: 10.2500/ar.2016.7.0150.
- Nelson HS, Lahr J, Rule R, et al. Treatment of anaphylactic sensitivity to peanuts by immunotherapy with injections of aqueous peanut extract. J Allergy Clin Immunol. 1997;99:744-751.
- Kim EH, Yang L, Ye P, et al. Long-term sublingual immunotherapy for peanut allergy in children: Clinical and immunologic evidence of desensitization. J Allergy Clin Immunol. 2019 Nov;144(5):1320-1326.
- Chinthrajah RS, Purington N, Andorf S, et al. Sustained outcomes in oral immunotherapy for peanut allergy (POISED study): a large, randomised, double-blind, placebo-controlled, phase 2 study. Lancet. 2019;394(10207):1437-1449.
- Bartlett JA, van der Voort Maarschalk K. Understanding the oral mucosal absorption and resulting clinical pharmacokinetics of asenapine. AAPS PharmSciTech. 2012;13(4):1110-1115.
- Passalacqua G, Villa G, Altrinetti V, et al. Sublingual swallow or spit? Allergy. 2001;56(6):578.
- Schneider H, Miller RK. Receptor-mediated uptake and transport of macromolecules in the human placenta. Int J Dev Biol. 2010;54(2-3):367-375.
- Samadi N, Klems M, Untersmayr E. The role of gastrointestinal permeability in food allergy. Ann Allergy Asthma Immunol. 2018;121:168-173.
- Li XM, Serebrisky D, Lee SY, et al. A murine model of peanut anaphylaxis: T- and B-cell responses to a major peanut allergens mimic human responses. J Allergy Clin Immunol. 2000;106:150-158.
- Eriksson K, Ahlfors E, George-Chandy A, et al. Antigen presentation in the murine oral epithelium. Immunology. 1996;88:147-152.
- Madsen C, Pilegaard K. No priming of the immune response in newborn brown norway rats dosed with ovalbumin in the mouth. Int Arch Allergy Immunol. 2003;130(1):66-72.
- Mondoulet L, Dioszeghy V, Ligouis M, et al. Epicutaneous immunotherapy compared with sublingual immunotherapy in mice sensitized to pollen (Phleum pratense). ISRN Allergy. 2012 Feb 2;2012:375735. doi: 10.5402/2012/375735. Print 2012.
- Zhang C, Ohno T, Kang S, et al. Repeated antigen painting and sublingual immunotherapy in mice convert sublingual dendritic cell subsets. Vaccine. 2014;32:5669-5676.
- Bagnasco M, Mariani G, Passalacqua G, et al. Absorption and distribution kinetics of the major Parietaria judaica allergen (Par j 1) administered by noninjectable routes in healthy human beings. J Allergy Clin Immunol. 1997;100:122-129.
- Teeguarden JG, Twaddle NC, Churchwell MI, et al. 24-hour urine and serum profiles of bisphenol A: evidence against sublingual absorption following ingestion in soup. Toxicol Appl Pharmacol. 2015;288:131-142.
- Xia B, Yang Z, Zhou H, et al. Development of a novel oral cavity compartmental absorption and transit model for sublingual administration: illustration with Zolpidem. AAPS J. 2015;17(3):631-642.
- Frischmeyer-Guerrerio PA, Keet CA, Guerrerio AL, et al. Modulation of dendritic cell innate and adaptive immune functions by oral and sublingual immunotherapy. Clin Immunol. 2014;155(1):47-59.
- Scurlock, AM. Oral and sublingual immunotherapy for treatment of IgE-mediated food allergy. Clin Rev Allergy Immunol. 2018;55:139-152.
- Canonica GW, Cox L, Pawankar R, et al. Sublingual immunotherapy: World Allergy Organization position paper 2013 update. World Allergy Organ J. 2014;7(1):6.
To view this issue and all back issues online, please visit www.drug-dev.com.
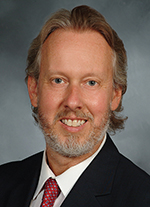
William Reisacher, MD, FACS, FAAOA, is a board-certified otolaryngologist, allergist, inventor, and Associate Professor at Weill Cornell Medicine in New York City. He has published and presented numerous chapters and research papers and served on the Board of Directors of the American Academy of Otolaryngic Allergy. Dr. Reisacher has founded companies in the biopharmaceutical, biotechnology, and digital healthtech spaces and lives in Manhattan with his wife and three children. Dr. Reisacher is an advisor and shareholder for both Allovate and Intrommune Therapeutics.
Total Page Views: 3947