Issue:April 2015
NANOSCALE PARTICLES - VAULT: A Novel Nanofrontier in Drug Delivery
ABSTRACT
Novel nanoscale particles (Vaults) as first described in 1986, exist in the multiples of thousands in most eukaryotic cells. Having an intricate shape composed of multiple curves evocative of cathedral vaults, therefore their name. The size, shape, eloquent assemblage, and molecular composition of vaults support their potential to be engineered into a delivery system for a broad range of therapeutics. Numerous strategies are under development to encapsulate chemically active small molecules, nucleic acids, immune modulators, and drugs into the vault particle. Vault nanocapsules also have the potential of being bioengineered to allow their use in a wide variety of biological applications, including drug delivery.
INTRODUCTION
Vaults are novel nanoscale particles that exist in most eukaryotic cells. They have an intricate shape composed of multiple arches reminiscent of cathedral vaults, hence their name. Vault size (~74 x 42 x 42 nm), shape, molecular composition, and facile assembly suggest they have the potential to be engineered to deliver a wide variety of therapeutics.1
Vaults were first seen while Rome et al were separating subpopulations of clathrin-coated vesicles using a preparative agarose gel electrophoresis technique and monitoring the fractions for purity using negative staining and transmission electron microscopy (TEM). Vaults were only visualized after isolation and negative staining, a method that reveals the structure of an object by exclusion of stain.2
STRUCTURE OF VAULTS
Determinations of mass by scanning transmission electron microscopy (STEM) confirmed that unstained specimens possess a two-fold symmetry; they contain two distinct and apparently equal centers of mass. The average mass of a single vault is 12,900 ± 1,000 kDa, a value consistent with the sedimentation behavior of vault particles on velocity sucrose gradients (approximately 150 S).3
COMPOSITION OF NATURALLY OCCURRING VAULTS
Because vaults were initially observed as contaminants in preparations of coated vesicles, numerous attempts were made to demonstrate a membrane within these particles. No vesicles could be observed by EM after removal of proteins by treatment with proteinase K or dissociation with 4.0 M urea; neither were phospholipids detected by biochemical techniques. Finally, these particles were found to be immunochemically distinct from coated vesicles. Thus, it appears that vaults are composed entirely of protein and RNA.2
DISTRIBUTION OF VAULTS
Vaults are highly conserved among vertebrate species and have been purified from the liver of chicken, cow, bullfrog (Rana catesbeiana), and the South African clawed frog Xenopus laevis, in addition to rat. However, vault concentration within different rat cell types shows marked heterogeneity. Although vaults present in all the cells, they are most abundant in macrophages and epithelial cells. Vault enrichment in microglia (brain macrophages) relative to other cells of the brain, allowed examination of the developmental profile of microglia in rat brain and provided new insights regarding the origin of these cells.4
VAULT OPENING: THE MECHANISM
The structure of the seven N-terminal repeat domains (R1–R7) that conform the vault waist is available at 2.1 Å resolution and accurately describes the interactions governing the association of the two vault halves. Analysis of contacts at the vault midsection reveals important charge complementarily at the interface of interaction between the two vault halves. R1-R1 contacts involve two R1 subunits in each half vault moiety. Among these contacts, the salt bridge formed between the strictly conserved residues Asp39 and Arg42 seems to be a key interaction.
Additional charged amino acids (Glu4, Glu5, and Arg37) and a cluster of hydrophobic residues (Ala6, Ile7, and Ile36), contacting through the two-fold axis of the particle, also contribute to the contact surface (Figure 1A). The observed interactions led to the proposal of a reversible mechanism of dissociation of the vault particle induced by a pH change. The low pH would facilitate disassembly of the particle by positive charge repulsion due to the protonation of the acidic residues at the interface. At higher pH, the aspartate and glutamate residues would recover their acidic state and re-establish the electrostatic interactions, permiting the re-association between the two vault halves. Afterward, the hydrophobic interactions would contribute to stabilize the locked conformation of the particle (Figure 1B).5
POTENTIAL APPLICATIONS OF VAULTS
Reversible pH Lability of Cross-Linked Vault Nanocapsules
The normal presence of vaults in humans at a copy numbers of over 10,000/cell makes them attractive as potential vehicles for drug delivery. Toward this target, bifunctional amine-reactive reagents are shown to be useful for the reversible cross-linking of recombinant vaults such that they may be closed and opened in a controllable manner.6 Yu et al studied the cross-linking of vaults with various agents and suggested that vaults may be engineered for reversible encapsulation of materials. For instance, vaults, which are natural residents of human cells, may be designed to carry genes or drugs prior to cross-linking, which will be delivered to a targeted site where the release of carrier molecule could be triggered by cleaving the vault cross-links.
Putative Cellular Functions
The fact that the murine MVP (Major Vault Protein) was found to be orthologous to the earlier described human lung resistance-related protein, known to be over expressed in multiple chemotherapy resistance models, immediately associated vaults with intrinsic drug resistance.7 This particle has also been implicated in the regulation of several cellular processes, including signal transmission, transport mechanisms, and immune responses.
The majority of reports agree that, at least in mammalian cells, vaults are predominantly (>90%) localized in the cytoplasm. Additionally, a subgroup of vaults was repeatedly reported to be detected in the nuclear envelope, suggesting that, at least occasionally, vaults play a role within the nuclear pore complex.8
Targeting of the Vault Nanoparticles to Specific Cell Surface Receptors
Kickhoefer et al carried out the study to target vault nanoparticles to specific cell surface receptors. These studies demonstrated that recombinant vaults assembled from MVPs containing C-terminal peptide extensions show these tags at the top and bottom of the vault on the outside of the particle, and that can be used to specifically bind the modified vaults to epithelial cancer cells (A431) via the epidermal growth factor receptor (EGFR), either directly (EGF modified vaults) or as mediated by a monoclonal antibody (anti-EGFR) bound to recombinant vaults containing the IgG-binding peptide. The aptitude to target vaults to specific cells represents an essential advance toward using recombinant vaults as delivery vehicles.9
Vaults Engineered for Nanobiotechnology
Ideally, pharmaceutical drug carriers should be biocompatible, should demonstrate prolonged circulation and protection of the encapsulated drug, and accumulate in the required pathological sites in the body. Various drug delivery and drug-targeting systems are currently under development. Their most important shortcomings include limited size, stability, or their inability to be targeted to specific tissues. Other protein assemblies, like viruses, have the major restriction of immunogenicity, limiting their applicability as drug delivery vehicles. Vaults, as naturally occurring particles, are non-immunogenic. This fact, coupled with their large size and the potential to encompass hundreds of proteins, have led to the suggestion that they could be utilized as natural nanocapsules for nucleic acid, drug, or protein delivery.10
Vault Nanocapsules With Fluorescent & Enzymatic Properties
Expression of MVP in insect cells, by the baculovirus expression system, exposed that this protein is only capable of directing the formation of recombinant vault particles with a structure similar to endogenous particles.11 Vaults can be engineered and expressed using a baculovirus expression system, and heterologous proteins can be encapsulated inside of these recombinant particles using a protein-targeting domain termed INT for vault INTeraction. Several heterologous proteins have been fused to the INT domain (eg, fluorescent and enzymatic proteins), and these fusion proteins when packaged into the recombinant vaults preserve their native characteristics, and consequently confer new vault properties.
Recombinant Major Vault Protein
Targeted to Neuritic Tips of PC12 Cells Herrmann et al investigated the targeting of recombinant major vault protein to neuritic tips of PC 12 cells grown in dimethyl ether supplemented with horse serum (10%) and fetal calf serum (FCS) (5%) at 37°C in the presence of 5% CO2. CHO and PC12 cells transfection with a cDNA encoding the rat major vault protein containing a vesicular stomatitis virus glycoprotein epitope tag demonstrates that the recombinant protein is sorted into vault particles and targeted like endogenous MVPs.12
In neuritic extensions of distinguished PC12 cells, there is a nearly complete overlap of the distribution of vaults and microtubules. A prominent co-localization of vaults with filamentous actin can be seen in the tips of neurites. Furthermore, in NGF-treated PC12 cells the location of vaults partially coincides with vesicular markers. Inside the terminal tips of neurites, vaults are located near secretory organelles. Their observations suggest that the vault particles are transported along cytoskeletal-based cellular tracks.12
FUTURE PROSPECTS
Nanoparticles called vaults, which are naturally present in human cells, may prove to be viable platforms for drug delivery. The fact that vaults can reversibly separate into two symmetrical halves has also been the focus of intense research. The possibility of closing and opening vaults in a controllable manner would be an attractive goal. A number of amine-reactive cross-linkers have been tested for their capacity to increase vault stability in order to prolong the half-life of the encapsulated drug.6 Another significant improvement would be to employ a combination of modified vaults with a variety of stabilities for the delivery of a specific drug.
REFERENCES
1. Rome LH. Vault nanoparticles: a platform technology for therapeutic delivery and vaccine development. Research Seminar Series. [Online]. http://keckmedia.usc.edu/mediasite/Viewer/?peid=c4278307e1ee4eca9b16f4982d930e4e. Accessed September 24, 2013.
2. Rome LH, Kedersha NL, Chugani D. Unlocking vaults: organelles in search of a function. Trends Cell Biol. 1991;1:47-50.
3. Kedersha NL, Heuser JE, Chugani DC, Rome LH. Vaults. III. Vault ribonucleoproteinparticles open into flower-like structures with octagonal symmetry. J Cell Biol. 1991;112:225-235.
4. Chugani DC, Kedersha NL, Rome LH. Vault immunofluorescence in brain: new insights regarding the origin of microglia. J Neurosci 1991;11:256-268.
5. Querol-Audi J, Casanas A, Uson I, Luque D, Caston JR, Fita I, Verdaguer N. The mechanism of vault opening from the high resolution structure of the N-terminal repeats of MVP. Embo J. 2009;28:3450-3457.
6. Yu M, Ng BC, Rome LH, Tolbert SH, Monbouquette HG. Reversible pH lability of crosslinked vault nanocapsules. Nano Lett. 2008;8(10):3510-3515.
7. Scheffer GL, Wijngaard PL, Flens MJ, Izquierdo MA, Slovak ML, Pinedo HM, Meijer CJ, et al. The drug resistance-related protein LRP is the human major vault protein. Nat Med. 1995;1(6):578-582.
8. Berger W, Steiner E, Grusch M, Elbling L, Micksche M. Vaults and the major vault protein: Novel roles in signal pathway regulation and immunity. Cell Mol Life Sci. 2009;66(1):43-61.
9. Kickhoefer VA, Han M, Raval-Fernandes S, Poderycki MJ, et al. Targeting vault nanoparticles to specific cell surface receptors. ACS Nano. 2009;3(1):27-36.
10. Kickhoefer VA, Garcia Y, Mikyas Y, Johansson E, Zhou JC, et al. Engineering of vault nanocapsules with enzymatic and fluorescent properties. Proc Natl Acad Sci. USA 2005;102(12):4348-4352.
11. Stephen AG, Raval Fernandes S, Huynh T, et al. Assembly of vault-like particles in insect cells expressing only the major vault protein. J Biol Chem. 2001;276:23217-23220.
12. Herrmann C, Golkaramnay E, Inman E, Rome L, et al. Recombinant major vault protein is targeted to neuritic tips of PC12 cells. J Cell Biol. 1999;144(6):1163-1172.
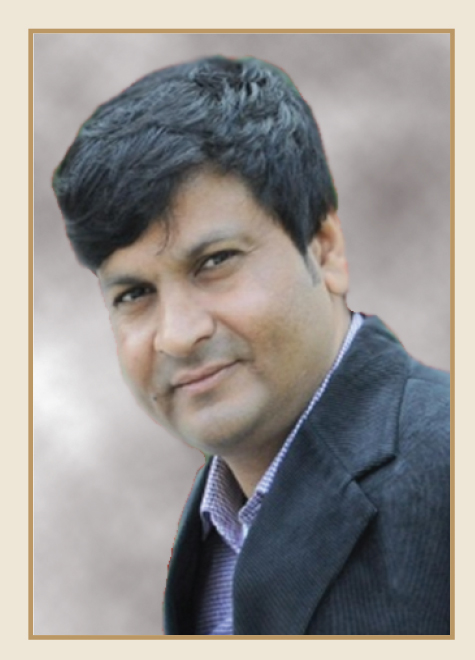
Dr. Jayvadan K. Patel is a Professor of Pharmaceutics and Principal, Nootan Pharmacy College, Visnagar-384315, Gujarat, India. He has more than 18 years of academic and research experience, has published more than 225 research and review papers in international and national journals, and has presented more than 150 research papers at various international and national conferences as author and coauthor. He has guided 26 PhD and 75 MPharm students for dissertation work. Dr. Patel is recipient of Fast Track Young Scientists Award by SERB (Department of Science and Technology), Government of India, New Delhi, recipient of a research grant under Research Promotion Scheme (Rs. 19.50 Lac) by AICTE, New Delhi, and recipient of the very prestigious award by APTI (Young Pharmacy Teachers of the Year-2014). He holds one patent, is serving as a peer reviewer for 26 well-reputed journals, and is serving as an Associate Editor/Member Editorial Board of 13 journals. Dr. Patel is also the recipient of Outstanding Young Indians-2010 award by JCI India and Young Pharmacist Award-2013 by Indian Pharmacy Graduate’s Association.
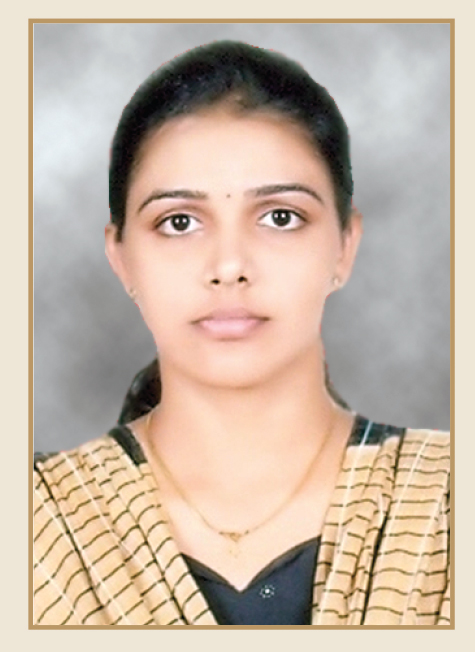
Dr. Anita Patel is currently working as Assistant Professor in the Pharmaceutics Department at Nootan Pharmacy College, Visnagar, India. She earned her Bachelors degree in Pharmacy from Gujarat University in 2002, her Masters degree in Pharmaceutics from Ganpat University in 2009, and her PhD in Pharmaceutical Science from Bhagwant University, Ajmer in 2013. Her research program is currently focused on application of novel nanofrontiers in drug delivery, nanobiomaterials for controlled drug delivery, drug nanocrystal technology, and artificial neural network in controlled drug delivery. She has published numerous scientific articles, conference abstracts, and book chapters in this area.
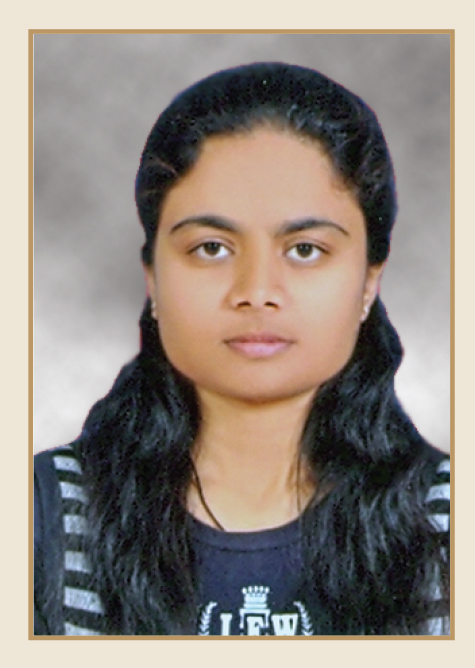
Ms. Vibha Champavat is currently working as Senior Officer-IRA in Regulatory Affairs Department at Claris Life science, Ahmedabad. Prior, she was worked as Assistant Professor in the Pharmaceutics Department at Nootan Pharmacy College, Visnagar. She earned her Bachelors degree from Shri Sarvajanik Pharmacy College in 2009 and her Masters degree in Industrial Pharmacy from Ganpat University in 2011. Her research program is currently focused on solubility enhancement, novel drug delivery systems, and nano-drug delivery systems. She has published numerous scientific articles, conference abstracts, and book chapters in this area.
Total Page Views: 2776