MULTI-PARTICULATE MANUFACTURING - How Does Experiment Design Affect Multi-Particulates Manufacturing?
INTRODUCTION
Multiparticulate dosage forms are gaining popularity and have many potential advantages over single-unit dosage forms, including reduced risk of dose dumping, increased bioavailability for compounds with short biological half-lives, predictable gastric emptying, less inter- and intra-subject variability, and better control over release patterns.1 Pellets are one of the most common of the various multi-particulate dosage forms. Pelletization is an agglomeration process that converts bulk powder or active pharmaceutical ingredient (API) blends with excipients into free flowing spherical or semi-spherical units, referred to as pellets, of desired size. Pellets range in size, typically, between 0.5 mm and 1.5 mm.1 Pellets as a drug delivery system offer not only the aforementioned therapeutic advantages, but also process advantages, for example, better flow properties, less friable dosage form, narrow particle size distribution (PSD), ease of coating, and uniform packaging. The reproducibility of the drug blood levels is an additional advantage with the use of a pellet formulation. Pellets are commonly filled into hard gelatin or hydroxypropyl methylcellulose (HPMC) capsules, but can also be compressed into tablets in some cases if desired.
Several factors affect drug product quality, inspiring the regulatory authorities worldwide to modernize good manufacturing practices such that quality is built-in by design, defining it as quality-by-design approach. Quality can be built into the drug product by comprehensive understanding of each unit operation in manufacturing as well as each quality attribute of the finished product, helping to gain understanding about the process as well as finished product during the development stage. The manufacturing process can be well understood when the target product profile is defined and the process flow is established after the initial development trials. Among the many development strategies, statistical design of experiments (DOEs) is considered the most beneficial tool for multi-factorial relationships investigations. Generally, for the test of k factors each at two levels, the factorial design requires 2k runs of experiments. As the number of factors or levels increases, the number of runs increases rapidly. Thus, it is advantageous to establish the critical process parameters (CPPs) that impact the drug product quality. The CPPs are parameters whose variability in limited range impact drug product critical quality attributes (CQAs) and hence should be monitored or controlled to ensure that the process produces finished product with acceptable quality. Statistical software packages are available to generate multi-level fractional factorial design of experiments, thereby reducing the number of experiments to be performed before obtaining an optimized range for the various CPPs selected. Sophisticated approaches to multi-particulate manufacturing can help ensure proper quality standards are met, while also speeding the development of innovative drug products. This is especially true as a growing proportion of small molecules in the drug development pipeline are being administered as combination therapies in which multi-particulate technology can play a role in development.
USING DOE TO ESTABLISH CRITICAL PROCESS PARAMETERS IN MULTI-PARTICULATE MANUFACTURING
Lonza researchers set out to investigate optimal multi-particulate manufacturing process design by focusing on a drug product containing a high drug load of a model water-insoluble [Biopharmaceutical Classification System (BCS) class 2 compound API]. The pellets were manufactured using the high shear granulation followed by extrusion spheronization techniques. This process involves wet granulation, extrusion, spheronization, fluid bed drying, and screening of dried beads through desired sieve stack to obtain the pellets of desired PSD.
Plackett-Burmann Design – DOE
This study was specifically designed to evaluate the impact of select processing parameters from the high shear granulation process toward the manufacturing of drug product, and their impact to critical quality attributes such as yield and dissolution. The data from this evaluation were designed to identify the processing parameters that need to be considered as CPPs and establish a proven acceptable range for each. All other manufacturing process parameters were constant for all the batches.
Several CPPs were identified based on prior knowledge of the unit operations involved, out of which three wet granulation CPPs were evaluated and optimized in the current study. Impeller speed, mixing time, and amount of binder solution used were identified as the process variables, whereas the percent yield and percent dissolution at 15 minutes were measured as response variables. The impeller speed was evaluated between 250-350 rpm, the mixing time was evaluated between 3-10 minutes, and the binder solution was evaluated in the range of 30%-45% w/w (Table 1).
Experiments were designed using the Plackett-Burman experimental design to evaluate the parameters. Plackett-Burman designs are usually resolution III, two-level designs. In a resolution III design, main effects are aliased with two-way interactions. The design selected was two-level factorial design for three factors with no center points or replicates. Table 2 represents the design of experiments generated by Minitab 17 following the Plackett-Burman half factorial design.
DOE runs 1-12 were executed, and the data generated were monitored for their effects on two critical quality attributes. First, the yield or the amount of beads generated in the acceptable particle size distribution range of 16 mesh to 30 mesh, and second, the amount of drug released within 15 minutes from the drug product generated in each experiment. Dissolution at t=15 minutes was monitored as part of heightened scrutiny of the processing parameters under consideration; however, the acceptance criteria is set at Q 75 in 45 minutes. This data was evaluated by plotting 2D contour plots using Minitab 17 software. Contour plots were formatted in accordance to the acceptability level of the critical quality attributes. The data were analyzed to evaluate the main effects as aliased with two-way interactions of the processing parameters. This information was used to decipher if a given processing parameter was considered a critical processing parameter and define a proven acceptable range for it. The optimized range obtained for the CPPs was further challenged for reproducibility as a similar batch size level as well as a 10x batch size scale up level. Thus, two additional batches were manufactured, one at 300-g level and another one at 3-kg level and evaluated for the CQAs to confirm the results obtained in this study.
RESULTS & DISCUSSIONS: IDENTIFYING EFFECTS OF SPECIFIC CPPS & PROVING BATCH REPRODUCIBILITY
Results of Factorial Design & DOE Analysis
This study identified the amount of binder solution as the most critical parameter that affects the dissolution profile from the granulated multi-particulate formulation. The dissolution profile was also affected by the mixing time and impeller speed when evaluated at constant amount of binder solution added. The yield was found to be significantly affected by a combination of all three variables. The current formulation presented differences in dissolution profiles with changes in the processing parameters, but passed the acceptance criteria of 75% (Q) in 30 minutes, therefore the ideal set-up for maximum yield was identified in the range of 275-300 rpm impeller speed with 8-10 minutes of mixing time and 42%-45% w/w binder solution.
Yield between 16/30 mesh as well as dissolution profiles for each DOE run 1-2 were obtained and evaluated. The current formulation presented differences in dissolution profiles with changes in the processing parameters (Figure 1), but passed the acceptance criteria of 75% (Q) in 30 minutes. Thus, dissolution at 15 minutes was used to evaluate the effect of process parameters. The yield values between 16/30 mesh as well as percent drug released at 15 minutes time point has been compiled for all DOE runs in Table 2. Contour plots as prescribed in Figure 2 were generated in Minitab 17 for each response factor versus each possible combination of the CPPs.
Response Factors Versus Amount of Binder & Impeller Speed
No significant effect of impeller speed on dissolution or yield for a constant amount of binder was observed in the test. Increasing the amount of binder improved the yield but was observed to slow down the dissolution. This could be explained by the increase in density of the granulation following increased amount of binder, which in turn can lead to denser extrudates and beads leading to slower dissolution rates. The increased yield could also be explained by denser granules and beads leading to a lower amount of fines being generated during spheronization as well as fluid bed drying process, increasing the amount of beads retained on 30-mesh sieve.
Amount of Binder
There was no significant effect of mixing time on the dissolution rate for a constant amount of binder. However, a mixing time of 8 minutes or higher favored a greater yield. The increase in amount of binder and mixing time from 30% w/w and 3 minutes to 45% w/w and 10 minutes, respectively, improved the yield from 40% to 72% (or higher). The increase in binder solution from 30% to 45% reduced the amount of drug released in 15 minutes from 100% to 60%, but was unaffected by the mixing time.
Response Factors Versus Mixing Time & Impeller Speed
Optimum dissolution was obtained with impeller speed between 250- 300 rpm and mixing times between 3-8 minutes. A maximum yield was obtained with a 250-300 rpm impeller speed and 8-10 minute mixing time.
Summary
For the formulation used in this study, the amount of binder was identified as the parameter with the most significant impact on CQA followed by mixing time and impeller speed. Combining the observations from the preceding discussion, the ideal set-up for maximum yield was identified in the range of 275-300 rpm impeller speed with 8-10 minutes of mixing time and 42%-45% w/w binder solution at 300-g scale.
MULTI-PARTICULATE PROCESS DESIGN IMPROVEMENTS CAN BE REPRODUCED & SCALED UP
Reproducibility Batch
The reproducibility of the manufacturing process was evaluated at a 300-g scale to verify the aforementioned design space using the optimized range of the parameters as mentioned in the summary. Maximum yield was obtained for this batch as compared to all of the DOE runs, which ascertains optimization and the accurate selection of the design of experiments. Refer to Figure 3 to see results obtained using the optimized CPP values in the verification batch at 300 g and scale-up batch at 3 kg.
Scale-Up Batch
A 10x scale-up batch was manufactured to evaluate the ability of design space to predict the scale-up parameters at 10x scale without impacting the CQAs. Yield as well as dissolution results obtained were in line with the results of smaller scale batches, further proving the reproducibility as well as the extension of the DOE run results to larger scale batches Refer to Figure 3 to see results obtained using the optimized CPP values in the verification batch at 300 g and scale-up batch at 3 kg.
CONCLUSION
Understanding the manufacturing process as well as finished product is of key importance in identifying the CPPs as well as CQAs for a multi-particulate drug product. High and low levels of CPPs (minimum and maximum settings of the operational ranges of CPPs) can be used to generate specification limits during the manufacturing process within which CQAs can be found to be acceptable. Operational ranges of critical parameters should be optimized in order to produce quality product in a repeatable manner. It could be concluded from the current study that the DOE approach can be used to optimize the critical process parameters that are reproducible at similar scales as well as 10 x scale-up. This study can be used as a guide in identifying an optimum range of CPP to be used during scale-up without impacting the CQAs. Additionally, it can be concluded that for the current process and the product, the amount of the binder solution had the maximum effect on the dissolution profile as well as percent yield.
These findings and other investigations into optimal process design may be of service to drug manufacturers seeking to rapidly advance new chemical entities from clinic to commercial stage. The careful application of design of experiment studies is an invaluable tool in proving the design space of complex formulations and manufacturing processes. Pharma & biotech companies developing combination therapies of incompatible APIs or modulated drug-release profiles can benefit from access to multi-particulate expertise, both in terms of product quality and faster development.
ACKNOWLEDGEMENTS
The authors acknowledge colleagues Nithin Vidiyala, Naveen Mangu, James Evans, Parag Ved, and Jeffrey Williamson for their efforts, contribution, and support provided in enabling this research.
REFERENCE
- Muley, S., Nandgude, T. and Poddar, S., 2016. Extrusion–spheronization a promising pelletization technique: In-depth review. Asian Journal of Pharmaceutical Sciences, [online] 11(6), pp.684-699. Available at: <https://www.sciencedirect.com/science/article/pii/S1818087616300721#ac0010>.
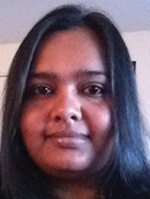
Namrata Vora is a Research Scientist in the Formulation Development group at Lonza Pharma & Biotech, Tampa FL, and has been with the company for over 8 years. Throughout her career, she has gained substantial experience of drug product development from early stage R&D through clinical development, FDA submissions, and lifecycle management. She earned her MS in Industrial Pharmacy from College of Pharmacy and Health Sciences, St. John’s University. Prior to that, she earned her BPharm from L.M. College of Pharmacy, Gujarat University, India.
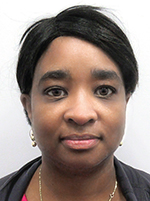
Danica M. Cartwright is a Research Scientist in the Formulation Development group at Lonza Pharma & Biotech, Tampa, FL. She has over 18 years of experience developing solid, oral dosage forms of pharmaceuticals from early stage R&D through clinical development. She earned her BS in Pharmaceutical Sciences from Campbell University.
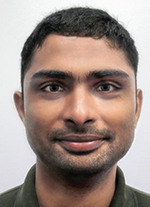
Karthikeyan Selvaraj is a Research Scientist at Lonza Pharma & Biotech, Tampa, FL, with a career in Research & Development for solid oral delivery systems. He earned his MPharm, with a focus in Pharmacology & Toxicology, from Long Island University, Brooklyn, NY. Prior to that, he earned his Bachelors from JSS College of Pharmacy, Ooty, India.
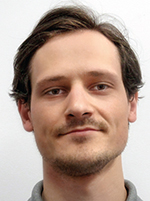
Ryan Larmon is a Research Associate at Lonza Pharma & Biotech, Tampa, FL. He is responsible for formulation and process development for solid oral dosage forms. He earned his MS in Pharmaceutical Sciences with a concentration in Pharmaceutical Chemistry from the University of Florida. Prior to that he earned his BS in Cell and Molecular Biology from University of South Florida.
Total Page Views: 4038