Issue:March 2021
MICROFLUIDIC ENCAPSULATION TECHNOLOGY - Achieving Reliable siRNA Drug Delivery for Inflammatory Diseases & Tumor Targeting by Nanoencapsulation
ABSTRACT
Nanoencapsulation is a highly efficient way to transport small interfering RNA (siRNA) therapies to targets across the cellular membrane, protecting the material from degradation prior to endocytosis. It is important that these therapies are delivered in a controlled and reproducible manner and, as the particle size affects the rate of uptake and clearance, consistent production of monodisperse particles is essential. The following discusses the benefits of microfluidic encapsulation technology for gene silencing applications in cancer immunology and inflammatory diseases, where siRNA can potentially be used to downregulate genes associated with these pathologies.
INTRODUCTION
The development of novel therapeutics for diseases that are difficult to treat or incurable is a major area of research, and RNA therapeutics are playing a growing role in new treatments. siRNA is a type of double-stranded, non-coding RNA molecule – about 20-25 nucleotides long – that can be used to downregulate the expression of genes via the RNA interference (RNAi) pathway. siRNA molecules have a 2-nucleotide overhang on the 3′ end of each strand and are bound by a multiprotein component complex known as RISC (RNA-induced silencing complex). The antisense single-stranded siRNA component guides the RISC complex to the target mRNA and aligns it. The catalytic RISC protein then cleaves the mRNA, inducing its degradation.1
RNAi offers one of the most promising areas for developing new treatments for a number of diseases since the discovery of siRNA in 1998.2 Using siRNA in this pathway opens up exciting possibilities in a breadth of applications, with each molecule capable of inactivating several target RNA molecules in a sequence-specific manner; siRNA doses of approximately 1-2 mg/kg2 can reversibly knockdown target protein production in vivo by up to 90%.3 With multiple siRNA treatments already in clinical practice, this method is gaining even more popularity, with ongoing research into chemical modifications aimed at improving qualities such as target cell delivery, siRNA potency, and serum stability, while reducing immunostimulation and off-target effects.4
THE FOCUS OF CURRENT RESEARCH
siRNA treatment is highly selective, and can be targeted at any gene involved with a specific disease. This makes it appealing for diseases with a genetic element or that are currently incurable by conventional drugs, for instance, cancer.
Multiple ongoing projects are looking to control general targets in certain diseases, such as the downregulation of TNF-alpha (TNFα) in active macrophages in rheumatoid arthritis, or the suppression of GATA-3 in T cells in asthma. siRNAs have also been developed to target oncogenes, as well as molecules critical for tumor-host interactions and tumor resistance to chemo- or radiotherapy.1,5 Treatment can even be personalized; a patient’s cancer can be sequenced to check for mutations to help discover the best siRNA sequence to use. For instance, EGF receptor mutations often cause chemo-resistance in lung cancer treatment with tyrosine kinase inhibitors, so these mutations can be targeted using siRNA. However, much of this research has been in vitro, using cell cultures – overcoming in vivo delivery difficulties is vital for widespread clinical use.
EFFECTIVE DELIVERY OF SIRNA THERAPEUTICS
Efficient delivery of siRNA treatments poses a huge barrier to wide-scale clinical use, as siRNAs must reach the cytoplasm of a cell to achieve post-transcriptional silencing. Although this represents one less barrier than those faced by plasmid delivery, which must reach the nucleus, efficiency of the delivery of free siRNA to target cells is commonly low as macromolecules cannot passively diffuse across cell membranes, and cells lack specific receptors for active nucleic acid uptake. Additionally, a large amount of siRNA is often degraded by nucleases in biological fluids.
There are several possible methods of in vivo delivery, including viral vectors, cationic lipids, polymer nanocarriers, and inorganic nanoparticles, each with their own benefits and limitations for therapeutic use. Research in the Merkel lab at the Ludwig Maximilian University of Munich is focused on novel non-viral and targeted nanosized siRNA delivery systems, using microfluidic mixing chips (Dolomite Microfluidics) for nanoencapsulation, to reliably and consistently produce monodisperse nanoparticles for targeted delivery of these therapeutics.
WHY NANOENCAPSULATION?
Nanoencapsulation is proving a highly efficient way to deliver siRNA to targets across the cellular membrane, protecting it from degradation prior to being taken up intracellularly by endocytosis. The actual sequence of the siRNA doesn’t have a big impact on particle characteristics, but it is important to deliver the therapy in a controlled and reproducible manner; particle size has a huge impact on in vivo work, affecting the rate of uptake and clearance.
Self-assembling polymeric nanoparticle systems are often prepared using a batch reactor technique. These polyplexes form by electrostatic interaction when using polyamine containing polymers that are mainly positively charged at physiologic pH. When mixed with negatively charged nucleic acids, nanoprecipitation occurs, resulting in the formation of polyelectrolyte complexes. This can be achieved by simply pipetting the polymers and nucleic acids together; however, such uncontrolled methods of mixing generate polydisperse nanoparticles with a broad size distribution. While this polydispersity often does not impact on in vitro research, it has a more relevant effect on in vivo models, where the production of monodisperse particles is key to delivery and uptake, and hence the success of any therapeutic.
In some circumstances, genes that are not the exact therapeutic target can also be affected by an siRNA sequence, causing off-target effects. The polymers and lipids used in nanoparticles can cause a variety of these side effects, such as complement activation or immunostimulation, and although some may be of benefit – depending on which immune pathways are activated – this can pose further difficulties. Treatment may also need to be specific to particular subpopulations of cells. For example, when treating rheumatoid arthritis by regulating TNFα, particles must be designed with properties that ensure they are only taken up by activated, and not resting, macrophages. Similarly, only activated, and not naïve, T cells should take up particles for asthma treatment, to prevent general immune suppression.
MICROFLUIDICS AS A METHOD FOR NANOENCAPSULATION
Microfluidic-based production of siRNA nanoparticles offers the reproducibility and consistency required for reliable drug delivery. Using microfluidics helps to regulate particle assembly; microfluidic devices allow control of the interaction of the cationic polymer with the anionic nucleic acid far better than batch methods, increasing colloidal stability. The constant ratio at which the polymer comes into contact with the nucleic acid increases particle uniformity, resulting in smaller, monodisperse particles with a much better polydispersity index and less batch-to-batch variability at scaled-up batch sizes. Additionally, microfluidic formulation of particles has been shown to yield more efficient gene knockdown in vivo than that from particles formulated in a batch reactor. This has been demonstrated in a lung model, where smaller particles with a lower polydispersity had much better gene-silencing efficacy of a housekeeping gene.2 It has also been noted that larger particles are phagocytosed more easily by macrophages, while smaller particles are a lot more efficient in epithelial uptake. A microfluidic production system can therefore improve a number of particle properties, and increase production scalability, which is a crucial consideration for any clinical trial.
THE IMPORTANCE OF MONODISPERSITY FOR NANOPARTICLE CHARACTERISTICS
Different characteristics and behaviors are preferential for different applications and can be controlled by the choice of nanomaterial. There is now an ever-expanding variety of particle formulations, taking advantage of different characteristics to improve delivery or carrier identity to achieve gene regulation. Nanomaterials that can be modified via PEGylation are frequently used to improve circulation time and decrease renal clearance for particles that are administered intravenously. PEG coatings help to shield the surface from aggregation, opsonization, and phagocytosis, all of which contribute to a longer systemic circulation time.6 Particles must also be altered to give the best possible chance of escaping the endosomal and lysosomal pathways. It is often the best route to look at hybrid materials and form particles from a combination of lipids and polymers. In this way, the best properties of polymers for shielding the particles can be combined with the fusogenic properties of lipids for the best intracellular delivery and endosomal escape. Using microfluidics enhances tweaking of particle characteristics, ensuring that particles are monodisperse and reproducibly produced, unlike batch methods that lead to the creation of irregular particles with different characteristics.
THE SIGNIFICANCE OF PARTICLE ASSEMBLY & SCALE-UP
Particle assembly has also been observed to play a key role in the efficacy of treatment, and the ability to efficiently scale this up is essential for widespread clinical adoption. The triblock copolymer polyethylenimine-polycaprolactone-polyethylene glycol (PEI-PCL-PEG) system can be modified to link targeting moieties, such as folic acid, to the micelleplex it forms with siRNA, and so microfluidic production of PEI-PCL-PEG nanoparticles enables rapid, scaled-up production of micelleplexes, while maintaining small and uniform particle distributions.7 These micelleplexes have been found to have superior delivery efficiency in comparison to polyplexes, due to their amphiphilic properties that aid cellular internalization and potentially endosomal escape.7 The system has been used to target folate receptor alpha (FRα), a tumor marker that is overexpressed in over 85% of all ovarian tumors, which shows real promise for potential treatment; folic acid can be attached onto the delivery vehicle, thereby mediating cell-specific siRNA delivery within tumors.
THE EFFECT OF MICROFLUIDIC PRODUCTION ON ADMINISTRATION
The administration route is another vital property for delivery of siRNA nanoparticles, as it is crucial that each treatment is taken up preferentially by the target cells. For instance, in order to reach specific cell populations within the lung or to deliver nucleic acids into the brain, targeting ligands can be attached to the surface of the delivery vehicle. These targeting moieties have a strong affinity with the target cells and tissues, and offer promise to detect distant targets, such as mobile immune cells, circulating tumor cells, or metastases.
Therapeutic nanoparticle administration routes include intravenous, transdermal, pulmonary, and intraocular options. Pulmonary delivery – inhalation of the particle formulation through the mouth so that the inhaled pharmacological agent reaches the lower airways – is one option, and may benefit treatments targeting local conditions in the lungs, such as asthma. Alternatively, intravenously administered nanoparticles are reported to accumulate in the liver – an ideal situation if the therapeutic target is in the liver. However, for targets located elsewhere, systemic administration isn’t always the best option to achieve the highest percentage of particle delivery, as the first-pass effect may reduce the amount of a drug delivered to a patient’s general circulation to small percentages of the total dose administered. Microfluidics offers a way to improve this situation, as the clearance by macrophages of smaller particles made using microfluidic assembly has been shown to be less efficient, decreasing the first pass effect.
TREATMENT LENGTH
As treatment is reversible, the length of effect of any siRNA depends on the biologic half-life of the target gene. For genes coding for a protein with a long half-life, often the effect of the siRNA silencing is delayed as an abundance of protein is still present. This means that treatment frequency can be anywhere from daily to weekly. But new methods are being developed all the time to improve dosing schemes, such as gel implants that degrade under certain physiological conditions – for instance, temperature or pH – to create a release-on-demand system for the siRNA particles. This can be applied to rheumatoid arthritis, where gels have been developed that are stable at physiologic pH and are expected to stay in the synovial cavity of the joint.8 When the pH drops due to inflammation, the gel starts to degrade, releasing particles to help fight inflammation. It is important for these particles to be monodisperse, so that siRNA release is controlled. However, much more research in vivo is needed to understand how long the implant would release an effective dose of the therapeutic.
SUMMARY
Optimizing particle characteristics, including formulation reproducibility, particle size, siRNA protection and release, toxicity, immunogenicity, and bioactivity, is critical for better in vivo results and to develop clinically relevant treatment forms, such as powders for inhalation with increased shelf-life.
Nanoencapsulation is proving to be a superior method for delivery of siRNA in vivo, and microfluidic methods allow precise control that is vital for efficient and effective production of smaller, uniform particles, ensuring a reliable and reproducible rate of uptake and clearance. This time-saving method offers improved batch-to-batch reproducibility, reducing the need for repeat experiments due to poor particle production, and production can be more easily scaled up for the clinical environment. Microfluidic encapsulation of siRNAs is therefore an efficient method for achieving reliable delivery of therapeutics for a whole variety of diseases. It is vital that research continues to improve and explore further applications for this technology, and it will be exciting to see where this leads in the future.
REFERENCES
- Dana H, Chalbatani GM, Mahmoodzadeh H, et al. Molecular Mechanisms and Biological Functions of siRNA. Int J Biomed Sci. 2017;13(2):48-57.
- Feldmann D, Xie Y, Jones S, Yu D, Moszczynska A, Merkel O. The impact of microfluidic mixing of triblock micelleplexes on in vitro/in vivo gene silencing and intracellular trafficking. Nanotechnology. 2017;28(22):224001.
- Zatsepin T, Kotelevtsev Y, Koteliansky V. Lipid nanoparticles for targeted siRNA delivery – going from bench to bedside. Int J Nanomedicine. 2016;11:3077-3086.
- Watts JK, Deleavey GF, Damha MJ. Chemically modified siRNA: tools and applications. Drug Discov Today. 2008;13(19-20):842-855.
- Feldmann D, Heyza J, Zimmermann C, Patrick S, Merkel O. Nanoparticle-Mediated Gene Silencing for Sensitization of Lung Cancer to Cisplatin Therapy. Molecules. 2020;25(8):1994.
- Suk JS, Xu Q, Kim N, Hanes J, Ensign LM. PEGylation as a strategy for improving nanoparticle-based drug and gene delivery. Adv Drug Deliv Rev. 2016;99(Pt A):28-51.
- Feldmann DP, Jones S, Douglas K, Shields AF, Merkel OM. Microfluidic Assembly of siRNA-Loaded Micelleplexes for Tumor Targeting in an Orthotopic Model of Ovarian Cancer. Methods Mol Biol. 2019;1974:355-369.
- Mohammadi M, Li Y, Abebe D et al. Folate receptor targeted three-layered micelles and hydrogels for gene delivery to activated macrophages. Journal of Controlled Release. 2016;244:269-279.
To view this issue and all back issues online, please visit www.drug-dev.com.
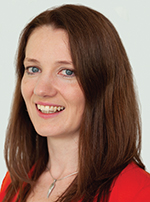
Dr. Olivia Merkel has been a Professor of Drug Delivery at LMU Munich since 2015. She is a registered pharmacist, having earned a Master’s degree in Pharmaceutical Technology in 2006 and a PhD in Pharmaceutical Technology in 2009, and has received numerous awards, including an ERC Starting grant, the APV Research Award, and the Carl-Wilhelm-Scheele-Award. She is the author of over 85 articles and book chapters, and has served as a reviewer for NIH (2014-2015) and SNF (since 2018). She is an Editorial Board member for JCR, EJPB, Molecular Pharmaceutics, and three other journals, as well as being the President of the German Controlled Release Society.
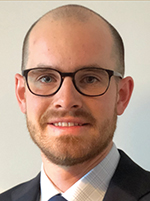
Christoph Zimmermann has been a PhD student in Professor Olivia Merkel’s group since February 2019. He is a registered pharmacist and earned a Bachelor’s degree in Management and Technology from the Technical University of Munich in 2013. He is a member of the German Pharmaceutical Society.
Total Page Views: 3610