Issue:May 2014
METAL-COORDINATED PHARMACEUTICALS - Reducing Inter-Subject Variability With Metal-Coordinated Pharmaceuticals: A Case Study With Furosemide
ABSTRACT
Oral furosemide, a loop diuretic commonly used to treat congestive heart failure and other fluid overload states, is poorly and variably absorbed, with bioavailability ranging from 11% to 90%.1 This variability can lead to unintended underdosing, re-dosing, and overdosing in both stable and deteriorating patients. Synthonics has used its metal coordination chemistry to create a novel coordination complex of furosemide and magnesium that is absorbed more efficiently and consistently than furosemide itself.
INTRODUCTION
Metal coordination entails attaching a minute quantity of a pharmaceutically acceptable metal, such as zinc, bismuth, or magnesium, to an active pharmaceutical agent to create a new and more effective molecule. By varying the metals and adjuvants used, metal coordination can affect a range of pharmacokinetic changes. For example, magnesium can improve a poorly absorbed drug’s solubility in water while maintaining the solubility in lipid bilayers necessary to permit its efficient passage from the digestive tract to the bloodstream and the target cell, while bismuth can impart bioadhesive properties to drugs that require controlled and extended release.
Although metal coordination can be used to impart a wide range of qualities to a diverse mix of drugs, this article focuses on its use to reduce the variability of absorption of furosemide. Furosemide is among the most commonly prescribed drugs for the management of congestive heart failure, hypertension, and other edematous states.2 Loop diuretics, such as furosemide, block the Na-K-Cl cotransporters in the ascending limb of the loop of Henle, which increases urinary sodium chloride and water excretion and induces a negative fluid balance in treated patients.3 They tend to have a relatively short duration of action and are typically utilized in more severe cases of fluid retention and congestive heart failure, and in chronic edematous states associated with renal insufficiency or hepatic dysfunction.4
Although furosemide remains the current standard of care for most patients, its erratic bioavailability can affect patients’ responses to daily furosemide dosing and can complicate the clinical management of patients receiving it in acute and chronic settings.5 Over time, the variability in absorption can lead to unintended under-dosing, re-dosing, and overdosing in both stable and deteriorating patients with inadvertent reflex-activation of the renin-angiotensin-aldosterone system, exacerbation of electrolyte imbalances, aggravation of diuretic resistance, and worsening fluid retention. Reducing the variability of furosemide’s absorption could significantly reduce the incidence of adverse effects and improve the clinical management of patients.
Synthonics has used its proprietary metal coordination chemistry to create a patent-protected magnesium-furosemide complex (Mg(fur)2, (Figure 1)) that enhances furosemide’s dissolution properties and improves its absorption.6
RESULTS
In vitro solubility and partition coefficient studies demonstrate the altered physicochemical properties of Mg(fur)2. The data in Table 1 show that complexing furosemide to magnesium increases its range of solubility (ie, amphiphilic properties). Of particular note are Mg(fur 2’s water solubility relative to magnesium furosemide salt and organic solvent solubility relative to furosemide.
In addition to increased solubility, Mg(fur)2 demonstrates more lipophilicity (greater log D) than fursosemide (Table 2). While enhancing lipophilicity through the reaction of a carboxylate with a metal ion might seem counter-intuitive, it is entirely consistent with the formation of a metal coordination compound with properties distinct from its metal salt. Thus, these data suggest a degree of covalency between the drug and the metal.
Mg(fur)2 dissolved much more rapidly inaqueous systems than did the magnesium furosemide salt. The magnesium furosemide salt had a solubility in the range of 5 to 10 mg/mL initially and remained in that range in water and simulated saliva (SS). In the fed state simulated intestinal fluid (FeSSIF), the concentrations of furosemide dropped from 5.3 to 0.6 mg/mL over 1 to 2 hours, possibly due to conversion of the salt form to furosemide and magnesium oxide. These solubility profiles contrast with that of Mg(fur)2, which rapidly dissolved (initial concentrations in the range of 32 to 52 mg/mL) and slowly converted to the salt (Figure 2).
Standard in vivo pharmacokinetic studies involving single oral doses of Mg(fur)2 and furosemide in a set of male Spargue-Dawley rats show the impact of Mg(fur)2’s physicochemical properties on the absorption of orally administered furosemide. As shown below, Mg(fur)2 was more rapidly and consistently absorbed relative to furosemide. This figure illustrates the plasma furosemide concentration versus time curves (plasma profiles) when rats (n = 5) were dosed with equimolar amounts (2 mg/kg furosemide) of standard furosemide, the magnesium furosemide salt, and Mg(fur)2.
Mg(fur)2’s enhanced and consistent absorption profile reflects the stability of the complex in the aqueous systems encountered between oral administration and initial interaction with the gastrointestinal epithelium. If hydrolysis of the complex had converted a significant amount to the salt, the plasma profiles would be more similar. Figures 4 and 5 show the plasma profiles of furosemide and the complex over three separate rat studies. In addition to the clear difference in the average Cmax of furosemide compared to the complex, the curves also indicate differences in inter-subject variability between furosemide and Mg(fur)2.
Although these data indicate that Mg(fur)2 is sufficiently stable in solution and in the GI tract, the precise kinetics of dissociation between magnesium and furosemide in the complex milieu of plasma and the GI tract are difficult to determine. Caco-2 studies and parallel artificial membrane permeability assay studies reveal no significant increase in permeability of Mg(fur)2 relative to furosemide and no meaningful change to the efflux mechanism. This may indicate that cell membrane components themselves cause dissociation of the magnesium furosemide coordination complex following administration.
Application of the Hartley test to the ratio of σ2 values in Table 3 indicates that the reductions in the variances were statistically significant at each time point with a confidence level of at least 95%.
Subsequent rat studies compared intersubject variability between Mg(fur)2 and furosemide. Furosemide (~10 mg/kg) andMg(fur)2 (~7 mg/kg) were fed to rats by oral gavage and blood samples were taken at the expected tmax for each test material and at a later time, tres, and the ratio of the variances between the two test materials at the two time points was calculated. The use of over 30 rats for each test compound assured statistically significant ratios. Based on earlier studies, tmax was estimated to be 90 minutes for furosemide and 20 minutes for Mg(fur)2. The tres values of 150 minutes for furosemide and 50 minutes for Mg(fur)2 were based on expected blood levels of about half Cmax.
The variability in the furosemide group was so large that tmax may have been anytime between about 90 minutes and 150 minutes. The consistency in the earlier animal studies with Mg(fur)2 indicated a tmax close to the predicted 20 minutes.
An χ2 test revealed that the standard deviation of absorption at the estimated tmax for Mg(fur)2 is approximately 2.4 times less than that of furosemide. Assuming that the reduction in variability at tmax persists throughout the drug’s absorption phase and extrapolating the results to humans, a patient taking a 40-mg pill could expect to absorb between 4 and 36 mg of furosemide (ie, 11% to 90% of the active agent), while one taking Mg(fur)2 could expect to absorb between 17 and 30 mg of furosemide (ie, 54% to 95% of the active agent).
The inter-subject variability of the furosemide dosed animals and the Mg(fur)2 dosed animals can be graphically represented by plotting the number of subjects against a range of plasma concentrations of furosemide at the estimated time of tmax. Figure 6 illustrates a normal distribution of absorption of furosemide at the estimated tmax in the population of animals dosed with Mg(fur)2 and a disparate and sporadically distributed absorption of furosemide at the estimated tmax in the furosemide dosed animal population, which indicates the significant reduction in inter-subject variability with Mg(fur)2.
DISCUSSION
There are several possible mechanisms responsible for transport of furosemide across the brush border membrane in the gastrointestinal tract. Whereas passive diffusion is reported to be furosemide’s principal mechanism of absorption, there is evidence for active transport mechanisms particularly associated with efflux, such as the p-glycoprotein system.7,8
The amino acid transport system relies on a sodium gradient when transporting bamino acids, such as furosemide. This sodium gradient causes the pH of the luminal side of the brush border membranes to be (5.5 to 6).9 If furosemide absorption depends, at least in part, on the sodium gradient, then the low pH microenvironment at the luminal surface of the intestines will reduce the solubility of furosemide, thereby limiting its availability for passive or active absorption.10 Mg(fur)2 is much more soluble than furosemide at this lower pH, and its dissociation rate is unlikely to be a factor during transport through the microenvironment. It seems plausible, if not likely, that the rate-limiting step for the absorption of furosemide is its movement through the low pH external surface layer lining the gastrointestinal epithelial cells. In addition, Mg(fur)2’s enhanced solubility and greater log D reveals an enhancement in the amphiphilic properties of furosemide. Amphiphilicity has been linked to improved bioavailability and enhanced penetration through the water/bilayer interface leading to improved adsorption at the gastrointestinal epithelia (Figure 7).12,13 The concomitant increase in solubility and enhanced amphiphilicity could translate into an overall improved bioavailability and faster onset of action. Figure 8 shows a three -dimensional plot of effective permeability (Peff), solubility (Dn), and relative bioavailability (BA) based on a well-known mathematical model.14
Key factors that influence the bioavailability of orally administered drugs include solubility and permeability. A significant component to a drug’s effective permeability is its availability for adsorption at the water/bilayer interface of the epithelial membrane. Therefore, for the purpose of this discussion, the relative Peff of Mg(fur)2 depends on its adsorption efficiency to the epithelial cell layer.
The plot in Figure 8 shows that at values of Peff and Dn along the steep slopes of the curve, changes in either factor or secondary effects, such as stress, edematous conditions, stomach contents, or gastric emptying, can cause a significant change in bioavailability. However, at the values of Peff and Dn represented by the plateau of the three-dimensional curve, changes in these factors cause little change in bioavailability. Thus, one can minimize the variability in a subject drug’s bioavailability by maximizing that drug’s absorption availability and solubility. Metal coordination chemistry provides the amphiphilic properties necessary to optimize both the absorption availability and therefore Peff and solubility, which is critical to maintaining bioavailability on the plateau of the three-dimensional curve.
Factors other than gastrointestinal epithelial permeability and solubility impact the inter-subject variability of furosemide (andother drugs for that matter). Still, the amphiphilic properties and enhanced dissolution of furosemide imparted through the application of metal coordination chemistry should result in a significant improvement in inter-subject variability.
To view this issue and all back issues online, please visit www.drug-dev.com.
REFERENCES
1. Murray, MD, Deer MM, Ferguson JA, Dexter PR, Bennett SJ, Perkins SM, Smith FE, Lane KA, Adams LD, Tierney WM, Brater DC, Open-label randomized trial of torsemide compared with furosemide therapy for patients with heart failure. Amer J Med. 2001;111(7):513-520.
2. Chui MA, Deer M, Bennett SJ, Tu W, Oury S, Brater DC, Murray MD. Association between adherence to diuretic therapy and health care utilization in patients with heart failure. Pharmacother. 2003;23(3):326-332.
3. Gheorghiade M. Treatment of congestion in acute heart failure syndromes: importance, strategies, and challenges. Amer J Med. 2006;119(12 Suppl 1):S1-2.
4. Gupta S, Neyses L. Diuretic usage in heart failure: a continuing conundrum in 2005. Eur Heart J. 2005;26(7):644-649.
5. Murray MD, Haag KM, Black PK, Hall SD, Brater DC. Variable furosemide absorption and poor predictability of response in elderly patients. Pharmacother. 1997;17(1):98-106.
6. Piccariello T. Metal Coordinated Compositions 7,989,440;2011. Available from: http://patft.uspto.gov/netacgi/nph-Parser?Sect1=PTO1&Sect2=HITOFF&d=PALL&p=1&u=%2Fnetahtml%2FPTO%2Fsrchnum.htm&r=1&f=G&l=50&s1=7,989,440.PN.& OS=PN/7,989,440&RS=PN/7,989,440.
7. Note that although the mean concentrations for the Mg(fur)2 group were lower than those of the furosemide group due to differences in dosage size, as a percentage of the administered dose, the mean concentrations for the Mg(fur)2 group exceeded those for the furosemide group.
8. Flanagan SD, Takahashi LH, Liu X, Benet LZ. Contributions of saturable active secretion, passive transcellular, and paracellular diffusion to the overall transport of furosemide across adenocarcinoma (Caco-2) cells. J Pharm Sci. 2002;91(4):1169-1177.
9. Pade V, Stavchansky S. Link between drug absorption solubility and permeability measurements in Caco-2 cells. J Pharm Sci. 1998;87(12):1604-1067.
10. Tsuji A, Tamai I. Carrier-mediated intestinal transport of drugs. Pharmaceut Res. 1996;13(7):963-977.
11. Daniel H. First insights into the operational mode of epithelial peptide transporters. J Physiol. 1997;498(Pt 3):561.
12. Balaz S. Lipophilicity in trans-bilayer transport and subcellular pharmacokinetics. Perspectives Drug Disc Design. 2000;19:157-177.
13. Balaz S, Lukacova V. Subcellular pharmacokinetics and its potential for library focusing. J Mol Graphics Modelling. 2002;20(6):479-490.
14. Oh DM, Curl RL, Amidon GL. Estimating the fraction dose absorbed from suspensions of poorly soluble compounds in humans: a mathematical model. Pharmaceut Res. 1993;10(2): 264-270.
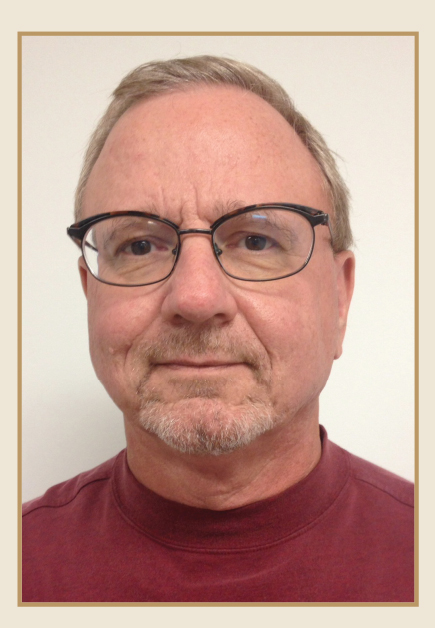
Dr. John D. Price is Vice President of Research and Development at Synthonics, Inc., where he is working on the design of new metal-coordinated pharmaceuticals, and on expanding this discovery platform into additional areas of drug delivery, including mucoadhesive bismuth coordination complexes for the controlled release of oral pharmaceuticals. Prior to joining Synthonics, Dr. Price worked in the fields of coordination and inorganic polymer chemistry as they relate to pharmaceutical development. As Director of Medicinal Chemistry at Allomed Pharmaceuticals, he led efforts investigating metal-coordinated insulin mediators as therapeutics for treating diabetes and Alzheimer’s disease. As Director of Research at Magnox, Inc. he helped develop several Super Paramagnetic Iron Oxide Nanomaterials. Dr. Price earned his BS in Chemical Engineering from Washington University in St. Louis and his PhD in Chemistry from Iowa State University. Over the course of his career, he has worked at the interface of several chemical disciplines, combining physical organic chemistry, synthetic biology, pharmacology, and metal coordination chemistry. He has publications and patents in all of these areas.
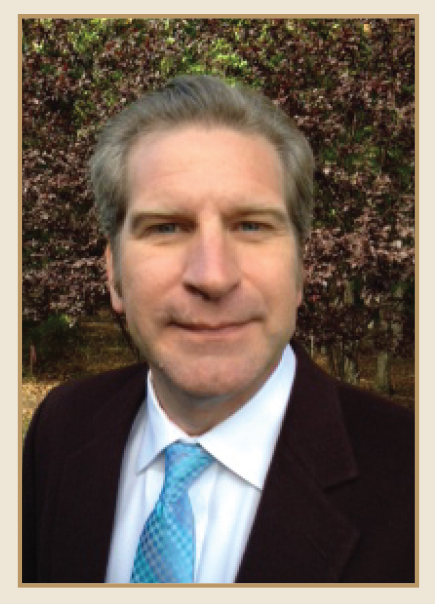
Dr. Thomas Piccariello is a Co-founder of Synthonics and currently serves as its President and Chief Science Officer. His current research is focused on the impact metal coordination has on pharmacokinetics and pharmacodynamics. Prior to founding Synthonics, Dr. Piccariello was Vice President of Polypeptide Drug Development at New River Pharmaceuticals and was the lead inventor on the Carrierwave technology. In that capacity, Dr. Piccariello oversaw the R&D efforts, including the set up of a cGMP CTM production facility. He has also been involved in the start-up of Insmed Pharmaceuticals. Dr. Piccariello founded (and later sold) Synthons, a CRO, and Chermetic, a contract manufacturing company. He earned his BS in Biology and his PhD in Chemistry from Virginia Polytechnic Institute and State University (Virginia Tech). Over the course of his career, he has worked in areas that involve synthetic chemistry, metallurgy, electrochemistry, plant enzymology, chemical manufacturing, pharmacology, and metal-coordination chemistry. Dr. Piccariello has over 100 patents and patent applications to his credit, most of which deal with novel drug delivery technologies.
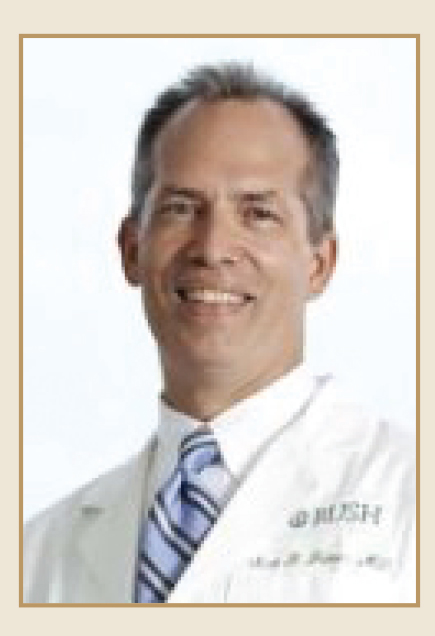
Dr. Scott Palmer, MD, is Chairman of the Scientific Advisory Board and a member of the Board of Directors of Synthonics, Inc. He’s a board-certified internist and an Assistant Professor in Department of Internal Medicine at Rush University Medical Center in Chicago. Dr. Palmer did his internship, residency and chief residency at Rush Presbyterian – St. Luke’s Hospital in Chicago and has been designated a top doctor by numerous regional and national publications. Dr. Palmer is a member of the American College of Physicians and is also a Medical Team Physician of the Chicago Bulls and the Chicago White Sox. Dr. Palmer’s research interests include pharmaceutical management of autistic spectrum disorders, cancer. He is a graduate of the University of Michigan and Rush Medical College and has an active clinical practice.
Total Page Views: 3540