Issue:November/December 2023
LIPOSOMAL PEPTIDE THERAPEUTICS - Revolutionizing Oncology: Unleashing Innovative Strategies to Tackle Challenging, High-Value Drug Targets
INTRODUCTION
The past 40 years in oncology have been dominated by the development of precision oncology approaches, or the idea that if one can identify the particular genetic perturbation that drives a patient’s cancer, one could design a medicine to target that particular mutation to stop cancer growth. The growth-promoting signals driving cancer proliferation flow from the cell surface through the cytoplasm to the nucleus, a type of oncogenic funnel so to speak (Figure 1), and as a field, we have attempted to stop that signaling flow by the development of monoclonal antibodies or small molecule kinase inhibitors to inhibit the cell surface receptors, such as the Epidermal Growth Factor Receptor (EGFR), or small molecule kinase inhibitors to block the activity of the cytoplasmic signal transduction molecules, like RAS or MEK, that carry those oncogenic signals from the cell surface to the nucleus.
While this approach has transformed the oncology space, significantly expanding our clinical toolkit and decreasing adverse effects for our patients, we now understand one of the liabilities of this approach. When we target the top or the middle of the oncogenic funnel, there is just too much residual opportunity for the tumor cell to evolve away from the blockade that was imposed on the cell. For example, Trastuzumab (Herceptin®), which targets one form of the EGFR oncogene (Her2) in breast cancer and one of the first targeted therapies approved in 1998, has transformed Her2+ breast cancer. In the metastatic setting, the addition of Trastuzumab to chemotherapy has increased overall survival (OS) to more than 4.5 years, compared to a life expectancy of 1.5 years seen in the 1990s. However, up to 30% of these patients develop resistance to these therapies, due to increased activation downstream of the imposed EGFR blockage. While efforts to develop better Her2-targeting therapies are ongoing, such as the approval last year of ENHERTU® (fam-trastuzumab-deruxtecan-nxki), these observations reinforce the idea we need to inhibit the bottom of the oncogenic funnel with more mutation-agnostic drugs, to inhibit the common factors at the end of the signal transduction cascade that drive the response to most if not all growth- promoting signals.5 Targeting downstream signaling proteins offers a promising approach to effectively mitigate the emergence of drug resistance that precision oncology therapies leave behind.
THE ONCOGENIC FUNNEL & CDK INHIBITORS
The bottom of the oncogenic funnel encompasses five cell cycle proteins that are members of the G1 CDK families (CDK4/6-cyclin D and CDK2-cyclin E) along with their unique regulator, p27Kip1 (Figure 1). Significant R&D resources have been dedicated to inhibiting CDK4/6 and CDK2, resulting in commercially successful CDK4/6 inhibitors (CDK4/6i), such as palbociclib, abemaciclib, and ribociclib.2 These are approved for treating metastatic hormone receptor+ (HR+) breast cancer and, more recently, as adjuvant therapy for high-risk non-metastatic HR+ patients. While these medicines have improved the progression-free survival rate, the development of resistance to these drugs remains a significant concern for almost all patients, resulting in a more modest OS and >40,000 patients in the US continuing to lose their lives to metastatic breast cancer each year.
The issue is that CDK4/6 is not the final player in the oncogenic signaling cascade. CDK2 can increase its activity and compensate for the loss of CDK4/6, driving the tumor out of remission and into a resistance state. CDK4/6 and CDK2 can be likened to a see-saw, where the effects of CDK4/6 on the left side can be counterbalanced by CDK2 on the right side. Therefore, instead of viewing CDK4/6 and CDK2 as distinct entities, it might be beneficial to think of them as a hub with the need to target both CDK4/6 and CDK2 simultaneously. Developing CDK2 single inhibitors (CDK2i) has presented challenges due to off-target toxicities, as the ATP binding pocket in CDK2 is structurally related to that of other essential CDKs, such as CDK1. Several promising CDK2i candidates, such as Pfizer’s PF-07104091, Blueprint Medicine’s BLU-222, and Incyclix Bio’s INX-315, are currently being investigated in Phase 1 clinical trials. Only time will determine if these novel CDK2i have achieved the necessary specificity for a favorable therapeutic margin. Notably, Nuvation Bio and Pfizer previously pursued the development of triple CDK4/6/2 inhibitors, but both of these programs were terminated due to unmanageable adverse effects.
P27 AS NOVEL TARGET FOR CDK INHIBITION
Concarlo Therapeutics has embraced a unique approach, by shifting its focus away from the conserved CDKs and instead targeting the master regulator of these kinases: p27 (Figure 2). p27 binds with high specificity to these complexes and ignores most of the rest of the kinome. Its endogenous function is to turn these kinases on and off, a function required during the cell’s natural cycling between proliferative and quiescent state. One way to think about p27 is to compare it to a door controlling access to activity of these kinases. When the key or the phosphorylation modification is present on p27 itself, the door swings open and CDK4/6 and CDK2 are on, but when the key is absent, the door slams shut, blocking the activity of these kinases. This dynamic opening and closing of the door is the way that CDK4/6 and CDK2 are regulated normally and p27 acts as the conduit between the left and right half of the see-saw, linking the activation or inhibition of CDK4/6 and CDK2 together.
We hypothesized that blocking the protein-protein interaction between p27 and its modifier Breast tumor-Related Kinase (BRK) would lock p27 into the door closed/off conformation. Furthermore, p27 is an intrinsically disordered protein, which means it only has structure when bound to its substrates, and traditional ATP-binding pockets, such as seen in kinases, are not present. Our hypothesis led us to develop a series of p27 inhibitors aimed at firmly shutting the door, effectively turning these three kinases CDK4/6 and CDK2 off in concert. By simultaneously inhibiting CDK4/6 and CDK2 via p27, our approach could address the tumor resistance seen in the presence of palbociclib, abemaciclib, or ribociclib. What sets our approach apart is that by targeting the bottom of the oncogenic funnel, our p27 inhibitors hold the potential to be more perturbation and tumor-type agnostic. This means they could effectively tackle resistance associated with various therapeutic antibodies against cell surface receptors, and the small molecule kinase inhibitors of the RAS/MAPK pathway, including Sotorasib, Cetuximab, etc. It is this innovative concept that gave birth to Concarlo Therapeutics, as we strive to harness the full potential of this groundbreaking approach.
THERAPEUTIC PEPTIDE FOR INHIBITION OF P27
Targets such as p27 may be more amenable to peptide approaches. Peptide drugs can effectively block protein-protein interfaces that are relatively featureless, can offer advantages related to specificity, and reduce the potential for off-target liabilities. Several high-value targets, such as Beta-catenin, Myc, or p53, have not been accessible by traditional small molecule approaches but newer traction has been achieved with peptide-based drugs (FOGPharma, Sapience Therapeutics, and PeptoMyc).
Initially, we took our lead from nature: a small protein called ALT (an alternative splice variant of BRK), which can bind to p27 and act like a blanket to block the door to keep it shut (Figure 2). In vitro, ALT expression resulted in its binding to p27 and inhibition of its phosphorylation, thus turning CDK4/6 and CDK2 off and blocking tumor cell proliferation. ALT cleared faster from non-tumor cells than from tumor cells, thus favoring longer inhibition of tumor cell- versus normal cell-proliferation. Expression of ALT in animal models of treatment naïve and CDK4/6i-resistant breast cancer caused tumor regression and increased OS. We screened variants of ALT based on an evolutionary informed approach of conserved sequence across species and nominated a lead therapeutic candidate peptide to progress into early nonclinical development. Additional optimization was undertaken, via protein engineering for modifications to enhance the peptide’s properties, including potency, selectivity, stability, manufacturability etc, and a lead peptide candidate, CCL20, was chosen for further development.4
One of the key challenges in manufacturing a peptide via chemical synthesis is both the cost and duration of the manufacturing process and low process yields, as well as the increased possibility of multiple process-related impurities. Concarlo approached this problem by investing in several proof-of-concept studies, comparing different peptide manufacturing techniques, such as Linear automated/manual Solid Phase Peptide Synthesis (SPPS), Fragment Condensation (FC) of several smaller peptide fragments, Native Chemical Ligation (NCL), and Chemoenzymatic Peptide Synthesis (CEPS) of main fractions using proprietary ligation conditions (Figure 3). One of the critical components of peptide manufacturing is the development of proper analytical tools for assessing peptide purity. While it is a common industry practice to use generic HPLC-based methods for assessing purity of novel peptides, in the case of Concarlo’s peptide, it became clear this approach (even coupled with Mass Spectrometry) was incapable of separating multiple smaller impurities and providing accurate purity readout. By switching to a UPLC method early in development and paying additional attention to in-process impurity characterization, Concarlo was able to significantly improve the overall purity of CCL20.
DELIVERY OF A THERAPEUTIC PEPTIDE TO TUMOR SITE
One of Concarlo’s challenges was delivery of its therapeutic peptide effectively to the tumor and into tumor cells, as the p27 protein is found intercellularly. Lipid nanoparticles (LNPs) are an attractive vehicle for delivery of different types of active pharmaceutical ingredients (APIs), such as small molecules, peptides, and nucleic acids.1 LNPs have a proven clinical safety record and have been successfully utilized in injectable formulations. Nucleic acid containing LNPs, similar to those used in COVID-19 vaccines, rely on delivering their cargo intercellularly by fusing with the target cell membrane and releasing their cargo into the endosome. This fusion is achieved through interaction of an ionizable cationic lipid of the LNP with the target cell membrane. These types of delivery systems are typically used for localized delivery (such as vaccines) or systemic delivery of gene therapies that benefit from ApoE-mediated uptake of lipoprotein particles. Delivery to extrahepatic tissues still remains one of the main challenges of LNP-based delivery systems.3 Conventional Liposomes, on the other hand, rely on the ability of small vesicles to extravasate at the tumor site following systemic administration, due to “leaky” tumor vasculature, that allows particles of up to 150 nm to penetrate into tumor tissue. This preferential accumulation of small LNPs into tumor has been called “enhanced penetration and retention” (EPR) effect, and in combination with long circulation times of liposomal drugs, was able to improve tumor delivery of known small molecule cytotoxic drugs, while reducing their known side effects. Since the approval of the first liposomal drug Doxil™ for breast cancer treatment in 1995, several other conventional liposomal drugs have been approved for other cancer indications (for example, Vyxeos® approved in 2017 for treatment of AML).
Concarlo’s approach to drug delivery is to utilize the best of both worlds by combining features of conventional liposomal formulations and nucleic acid LNPs. We need to ensure our delivery vehicle is able to fuse with the tumor cell membrane and release the cargo intercellularly. Cationic lipids historically used for nucleic acid delivery have been known to promote membrane fusion and allow for efficient transfection of the target cells. Although Concarlo’s peptide is significantly smaller than mRNA, it is as highly negatively charged as nucleic acids and relies on the same intercellular delivery principles as nucleic acids do. During liposomal particle formation, it complexes with the cationic lipid, and this charge/charge interaction ensures high loading efficiency of the peptide into the lipid nanoparticle. The presence of other lipids in the formulation, such as cholesterol helper lipid and PEG lipid, ensures both stability and long circulation half-life of peptide- containing liposomes.
MANUFACTURING OF LIPOSOMAL PEPTIDE THERAPEUTIC CANDIDATE
The ability to scale-up production of liposomal formulations remains one of the key aspects of successful liposomal product development (Figure 4). Although the advancement of off-the-shelf microfluidic instruments has enabled research scientists to produce small batches of liposomal formulations with relative ease, further scale-up required to support preclinical and clinical product demands can pose a significant challenge. Typical steps required for LNP manufacturing include LNP formation, solvent removal by buffer exchange, and product concentration by ultrafiltration, followed by aseptic filtration through 0.2-μm sterile filters. The LNP formation step involves microfluidic mixing of solvent stream containing lipids with aqueous stream containing the payload, such as peptide, small molecule, or nucleic acid. Consistent and reproducible LNP formation across different scales is the key to successful scale-up of LNP formulations, as this step controls both the particle size and encapsulation efficiency of the resulting LNPs. Typically, conditions for LNP formation do not scale-up linearly, and therefore, conditions developed during discovery phase using laboratory scale equipment likely will not be directly transferable to a larger scale production.
WHERE ARE WE NOW WITH CMC PROCESS & PRODUCT DEVELOPMENT?
At Concarlo, we successfully manufactured our peptide drug substance as well as our liposomal formulation using a scalable and controlled process. We were able to establish feasibility of several manufacturing routes for production of our peptide. We have invested in extensive physical/chemical characterization of key peptide properties, such as solubility, conformation assessment, and salt form impact. We transitioned from a laboratory scale process for making LNPs to an industry-standard process capable of supporting clinical development. We are currently gearing up to optimize our liposomal formulations for the best encapsulation efficiency, strict particle size specifications, and optimal stability to support nonclinical studies. The extensive in vitro screening of the formulation variants for enhanced cellular uptake of our therapeutic peptide would allow further improvement in in vivo efficacy and tolerability. Simultaneously, we developed key phase-appropriate analytical assays for lipid and peptide analysis to support our future IND-enabling stability program. We are scaling up the manufacturing process of our drug product in preparation for in vivo pharmacokinetic/pharmacodynamic testing and indication expansion while supporting an accelerated nonclinical safety assessment toward submission of an IND application for initiation of first-in-human Phase 1 clinical trials.
Rather than persistently seeking a me-better variation of existing targets at the upper or middle regions of the oncogenic funnel, we hope the field will continue to exploit novel approaches to target intracellular proteins that are known high-value oncology targets at the bottom of the oncogenic funnel. Moreover, the oncology field could draw inspiration from the vaccine and gene therapy sectors and embrace innovative strategies in manufacturing formulations that enable the systemic and safe delivery of peptides across the cell membrane and efficient release of peptides from the endosome to maximize their intracellular effectiveness. This cross-pollination of knowledge between fields has the potential to revolutionize the delivery of therapeutic peptides in oncology, fostering advancements and offering new possibilities for effective treatment options. Through our commitment to innovation and scientific excellence, we invite potential development partners to join us in our pursuit of advancing liposomal peptide therapeutics and making a positive impact on patients’ lives.
REFERENCES
- Cullis PR, Hope MJ. Lipid Nanoparticle Systems for Enabling Gene Therapies. Mol Ther. 2017 Jul 5;25(7):1467-1475. doi: 10.1016/j.ymthe.2017.03.013. Epub 2017 Apr 13. PMID: 28412170; PMCID: PMC5498813.
- Goel, S., Bergholz, J. S., Zhao, J.J. Targeting CDK4 and CDK6 in cancer. Nat Rev Cancer. 2022 Jun;22(6):356-372. DOI: 10.1038/s41568-022-00456-3.
- Hou, X., Zaks, T., Langer, R. et al. Lipid nanoparticles for mRNA delivery. Nat Rev Mater 6, 1078-1094 (2021). https://doi.org/10.1038/s41578-021-00358-0.
- Jilishitz, I. et al. NP-ALT, a Liposomal:Peptide Drug, Blocks p27Kip1 Phosphorylation to Induce Oxidative Stress, Necroptosis, and Regression in Therapy-Resistant Breast Cancer Cells. Mol Cancer Res (2021) 19 (11): 1929-1945.
- Mendes, D., Alves, C., Afonso, N. et al. The benefit of HER2-targeted therapies on overall survival of patients with metastatic HER2-positive breast cancer Breast Cancer Res. 2015 Nov 17;17:140.doi: 10.1186/s13058-015-0648-2.
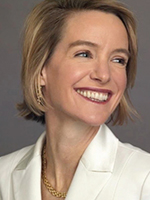
Dr. Stacy Blain is Co-Founder and Chief Scientific Officer of Concarlo Therapeutics. She is an internationally known expert in cell cycle and cancer biology, and is one of the world’s experts on p27Kip1. She has been studying cell cycle regulation for more than 25 years as an NIH-funded investigator and tenured Associate Professor at the SUNY Downstate Medical Center. She founded Concarlo, capitalizing on discoveries she made and patented. She was trained at Princeton, Columbia, and the Memorial Sloan-Kettering Cancer Center.
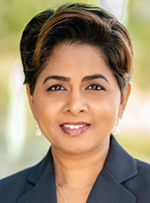
Dr. Krishna Allamneni is Chief Development Officer at Concarlo Therapeutics. She is a biopharma R&D expert with almost 20+ IND/IMPD submissions, 6 NDA/MAA approvals, and a track record of efficiently developing therapeutic candidates of various modalities. A forward-thinking leader in R&D strategy and tactical planning, and a highly regarded operational manager for key R&D functions, she has impactful global regulatory interactions with FDA, MHRA, BfArM, ANSM, PMDA, Health Canada, TGA etc. during clinical development, partnership, and commercialization. She was formerly with Turning Point, Jazz Pharmaceuticals, and Genentech.
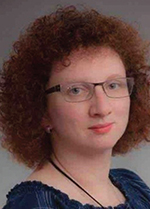
Natalia Zisman is Director, Chemistry, Manufacturing and Controls at Concarlo Therapeutics, specializing in liposome and lipid nanoparticle drug delivery systems. Prior to her current role, she held various positions at Evonik Vancouver laboratories, facilitating transition of customer projects through various stages of development, from proposal scoping to formulation development to early clinical manufacturing. She has over 20 years of experience in the lipid nanoparticle drug delivery field and has been involved in all aspects of liposome manufacturing, starting from early stage formulation design, process development, scale-up, and early clinical manufacturing. Prior to Evonik, she worked at a number of Vancouver-based drug delivery companies (CDRD/adMare, Celator/Jazz, Inex), focusing on liposomal products. She earned her undergraduate degree in Chemistry from University of British Columbia.
Total Page Views: 3274