Issue:September 2022
GENE THERAPY - Developing Affordable Point of Care CAR-T Therapies: Expanding Efficacy & Impact
INTRODUCTION
The hypothesis that engineered T cells could recognize and eliminate hematologic malignancies has proven true. The most recent headlines in which two of three chronic lymphocytic leukemia (CLL) patients treated with an anti-CD19 CAR-T cell product remain disease free 10 years after treatment continue to affirm the durable efficacy of CAR-T cell therapy.1 At the most recent annual meeting of the American Society for Hematology (December 2021), major sessions were devoted to the question as to when CAR-T cell therapy could be moved up to be a second line therapy in adults with B cell leukemia and lymphoma.2 This would significantly expand the pool of patients eligible for this therapy.
WHAT WE KNOW
A CAR (Chimeric Antigen Receptor) is a cell surface protein expressed on the surface of a cell, introduced by a gene vector. CARs can be expressed on T lymphocytes (T cells), NK (natural killer) cells, and macrophages. T cells exhibit a broad range of activity.
The T cells engineered by means of a gene vector to express a CAR (CAR-T) were initially intended to exert immune effects designed to eliminate cancer cells. However, the field of use has broadened to include the engineering of T-regulatory cells and T cell populations that can remodel tissue. T-regulatory cells can reverse inflammation or auto-immunity in serious human diseases, such as diabetes and rheumatoid arthritis, and also facilitate organ engraftment. Thus, a number of companies focused on CAR-Treg have been founded or acquired CAR-Treg assets, including Sangamo, Sonoma Biotherapeutics, GentiBio, and Abata Therapeutics.
In a remarkable new application, CAR-T cells specific for FAP (fibroblast activation protein, expressed on activated fibroblasts) were shown to reduce cardiac fibrosis in an animal model.3 This represents a third field of use for CAR engineered T cells, wherein the therapeutic goal is to alter the cellular composition of a targeted tissue.
The primary domain of activity for CAR-T remains hematologic malignancies. All of the currently approved products target either the CD19 protein expressed on leukemia and lymphoma or the BCMA protein, expressed on multiple myeloma. These are: Abecma (idecabtagene vicleucel for multiple myeloma), Breyanzi (lisocabtagene maraleucel, for diffuse large cell B cell lymphopma, DLBCL), Carvykti (ciltacabtagene autoleucel, for multiple myeloma), Kymriah (tisagenlecleucel, DLBCL and pediatric acute lymphocytic leukemia, ALL), and Tecartus (brexucabtagene autoleucel, mantle cell lymphoma)/Yescarta (axicabtagene ciloleucel, DLBCL and follicular lymphoma). The final two listed are essentially the same anti-CD19 CAR-T, but with different applications and slightly different manufacturing, one of which features a T cell enrichment step.
COST OF CARE PROHIBITS WIDE SPREAD USE
One of the major critiques of the currently approved CAR-T cell products is their cost. Drug acquisition costs range from $373,000 to $475,000 and represent the largest component cost of the therapy. A real-world estimate of total costs places the average at $700,000 and can exceed $1 million in some cases (Aislinn Antrim, Pharmacy Times, 2021).
How did we arrive at this astronomical price tag? In past approaches, complex therapeutics in which cells were removed from the body and selected or genetically engineered, the work was carried out in academic medical centers using reagents and devices that were clinically safe, but not specifically designed as an all-encompassing GMP product. The many different approaches to bone marrow transplantation, implemented at numerous cancer centers, had been the working model for cell and gene therapy to this point. As the process of creating a CAR-T cell generation became more refined and reproducible, pharmaceutical companies stepped in and created a pathway to generate autologous cell-based therapeutic products.
While a boost to the field with regard to interest from investors, there are drawbacks. By adopting a large-scale central manufacturing model, new innovations are potentially stifled, as corporations have to recover the extraordinary costs devoted to creating what is essentially a first-generation product. Moreover, these first-generation products are not as efficient or effective as the T cells bearing the CAR are over-activated and differentiated, leading to decreased efficacy in the body.
FINDING A BETTER WAY
The following presents three pillars by which efficacy and impact can be increased, and by which costs can be significantly lowered, leading to greater availability of CAR-T products for all those who would benefit. The first is using a place-of-care method for CAR-T production, the second is the advent of extremely short CAR-T generation protocols, and the third, while still on the horizon, has the ability to upend the entire field. Generation of CAR-T by direct injection of gene vector particles into the body, allowing for transduction, activation, and expansion CAR-T, would entirely alter how we approach cell and gene therapy.
PLACE-OF-CARE MANUFACTURING FOR CAR-T
The current approved therapies for CAR T cell production require extensive logistical and regulatory support. When patients are approved to receive CAR-T cell therapy, they first undergo apheresis (leukocytapheresis/leukopheresis), a procedure whereby a patient is connected to a medical device that receives whole blood from a vein, the white cell fraction of the blood (leukocytes) is harvested by means of differential centrifugation, and the red cell fraction returned to the patient. Apheresis is a costly step requiring dedicated personnel and devices. The advantage is that far more white blood cells (lymphocytes) can be collected than from a normal blood draw. Once collected, the leukocytes are processed, frozen, and then shipped to a central manufacturing facility. There, the cells are thawed, processed, and transduced with a retroviral or lentiviral gene vector. Once transduction has taken place, and the engineered cells pass release criteria (such as sterility and evidence of CAR expression), they are repackaged and shipped to the clinical site for infusion into the patient.
Caring Cross proposes that the economics of place-of-care manufacturing will continue to grow in importance, both in the current US market, and across the world. The argument or challenge against place-of-care manufacturing usually centers on reproducibility. In order to demonstrate that place-of-care manufacturing can be robust and reproducible, we tested CAR-T production in two geographically disparate sites using identical manufacturing platforms, reagents, and lentiviral vector (LV).
Very similar CAR-T were manufactured and used to treat patients at both Case Western Reserve/University Hospitals Medical Centers in Cleveland, OH, and the Dimitry Rogachev Pediatric Cancer Hospital in Moscow, Russia. These place-of care products were generated in a much shorter time than commercial products and were used to treat patients who had more severe disease (and could not wait the weeks required for central manufacturing of CAR-T).4 Our experience with other sites employing place-of-care manufacturing show similar high-quality products being produced with exceptional outcomes.5 Using a closed system, the cell manufacturing steps in these trials were carried out in the bone marrow transplant laboratories, and thus in a controlled environment, but outside of a traditional clean room. This has great implication for cost savings. Using the closed manufacturing devices, currently available on the market, allows a center to operate with minimal reliance on a clean room suite, Figure 1.
The use of place-of-care manufacturing has been essential in the manufacturing of CAR-T cell products outside the US. Not waiting for costs to drop on their own, Spanish investigators formed a national network, generated their own LV, and a employed a commercial cell production platform (CliniMCAS Prodigy, Miltenyi Biotec). Hospitals were able to produce CAR-T products for B cell malignancies at a greatly reduced cost that had similar clinical benefit as current commercial products.6 National healthcare systems across the globe are taking note of this approach, and it will surely expand. The UK government has collected comments and will soon issue guidance for place-of-care manufacturing.7 This will establish the needed regulatory framework and potentially place the UK in the lead for both CAR-T production and innovation.
SHORT CAR-T GENERATION PROTOCOLS
The creation of CAR-T cell products outside the body is a complex and lengthy process. Putting the logistics of where the CAR-T cells are produced aside, the manufacturing process can be 8 days or longer. At the production facility, the CAR-T cell production process starts with the isolation of either total leukocytes (PBMC) or selection for CD4 and CD8 T cells by immunomagnetic bead selection. Isolated T cells are then activated with reagents designed to cause cell activation and expansion (ie, Dynabeads CD3/CD28 CTS™, Invitrogen; T Cell TransACT™, Miltenyi Biotec; GMP Cloudz T Cell Activation Kit, R&D Systems). This set-up process usually takes a single day, followed by transduction with a lentiviral or retroviral gene vector that encodes the CAR. This adds another 2-3 days. The subsequent 5-9 days of culture allows for CAR-T to expand to sufficient numbers for formulation for either infusion or cryopreservation. The media and supplements (including cytokines like IL-2, IL-7, IL-15, or IL-21) must also be clinical grade and add to the costs.
Regulatory agencies require some evidence that the T cells treated with a gene vector do not carry along with them sufficient amounts of plasmid used during production of the gene vector (usually a PCR-based test) to be expressed in the host. Also the product is tested for the absence of replication-competent virions (termed RCL, replication competent lentivirus) arising from recombination of genetic elements. While RCL has never been observed in a clinical process, samples must at least be retained in order to allow for subsequent testing.
Investigators have been working to decrease the culture time following transduction with LV. The barriers have been both regulatory and methodological. The regulatory hurdle seeks to quantify the amount of residual elements used during CAR-T manufacture that are carried forward into the infusion product. The methodological challenge is to prove that the CAR is indeed now being expressed by the transduced T cell population. In addition to these concerns, it is well recognized the longer T cells are cultured the more “differentiated” they become. A differentiated T cell has decreased ability to expand and exert activity against diseased tissue.
A recent publication from Dr. Milone’s lab at the University of Pennsylvania took all of these challenges straight on and created a single-day CAR-T generation process that is not dependent on the addition of a T cell stimulation reagent.8 Although, a regulatory path forward has yet to be determined, the methodological barriers to ultra-rapid CAR-T production are falling rapidly. The ability to create a CAR-T product in a single day renders the complexities of a central manufacturing approach essentially a moot question. It is no longer worth the trouble.
DIRECT IN VIVO APPLICATION OF GENE VECTORS TO PRODUCE CAR-T
Given the onset of ultra-rapid generation of CAR-T outside the body, the next logical step is to do away with cell processing outside the body entirely, and to directly inject gene vectors (Figure 2). For such a vector to be successful, it would need to have both selective targeting/transduction of the intended T cell population, as well as some mechanism to expand or select for transduced cells once the gene vector has been administered.
A recent entrant to the field, Umoja Biopharma, is proposing to generate CAR-T with a vector particle that can be directly injected to the body, and which binds to target T cells by virtue of a specific receptor in the particle outer membrane. In addition to a CAR, the payload within the gene vector includes a rapamycin-activated signaling protein that initiates IL-2 like signaling in the transduced T cells. Rather than encoding a “driver” like signaling protein, companies like Enochian BioSciences endow their gene vector with a drug-resistance gene, in this case to the drug cyclophosphamide. Cyclophosphamide is used in CAR-T therapy to reduce endogenous immune cells and allow for CAR-T expansion. Enochian will take advantage of this process by using the drug for in vivo selection of transduced cells.
While targeting the LV to the right T cells and providing for a method of selection or expansion is essential, one more barrier may yet remain. It appears the most common envelope protein (the protein on the lentiviral vector surface that allow for entry into the targeted cell) VSV-G, can be inactivated by serum complement. Fortunately, researchers at the Fred Hutchinson Cancer Research Center have developed a new envelope protein that can be used instead of VSV-G, derived from the Cocal virus.9 Thus, use of Cocal envelope protein in lentiviral vector production, combined with preferential targeting and selection, will soon bring the era of direct LV transduction in the body to pass.
Given the rapid development of the field, other instantaneous methods of gene transduction, such as electroporation with Crispr-based gene modification, will also play a role but will likely remain a process carried out on cells outside the body.
SUMMARY
The commercialization of CAR-T manufacturing was a watershed moment in cell and gene therapy. What was once a process limited to highly skilled and resource-rich academic medical cells was now made available to any medical center with cell therapy experience, essential a center THAT has a track history in bone marrow transplantation. However, the high price for central manufacture of CAR-T has important limitations to its long-term use. The high price for CAR-T products makes them unavailable in low- and middle-income countries, and for individuals with inadequate healthcare coverage in high-income countries.
This represents a serious justice issue. These therapies were developed using many public resources (such as NIH-funded comprehensive cancer centers, and IP developed in public institutions). If the benefit of these therapies are available only for the economically elite, public trust in medical science will be undermined. In addition, while the learning required to create a commercial product featuring genetically engineered cells was impressive, the costs to setting up these systems creates a competitive landscape against new innovative technologies that could drive down cost. And finally, the technology and the regulatory framework required to safely oversee the development of CAR-T therapy continues to evolve.
The future belongs to those who will be able to innovate rapidly, maintain regulatory confidence, and drive down costs in order to make CAR-T cell and other engineered cell therapies available to all who would benefit.
REFERENCES
- Melenhorst JJ, Chen GM, Wang M et al., Decade-long leukemia remissions with persistence of CD4+ CAR T cells. Nature 602:503-509 (2022). doi.org/ 10.1038/s41586-021-04390-6. PMID: 35110735.
- Locke FL, Miklos DB, Jacobson C, et al. Primary analysis of ZUMA-7: a phase 3 randomized trial of axicabtagene ciloleucel (axi-cel) versus standard-of-care therapy in patients with relapsed/refractory large B-cell lymphoma. Abstract #2. Presented at the 2021 American Society of Hematology Annual Meeting, December 12, 2021.
- Rurik JH, Tombacz I, Yadegari A, et al., CAR R cells produced in vivo to treat cardiac injury. Science 375:91-96 (2022). doi.org/10.1126/science.abm0594. PMID: 35990237.
- Maschan M, Caimi PF, Reese-Koc J, et al., Multiple site place-of-care manufacturied anti-CD19 CAR-T cells induce high remission rates in B-cell malignancy patients. Nature Communications 12:7200 (2021). PMID: 34893603.
- Hah N, Johnson BD, Schneider D, et al. Bispecific anti-CD20, anti-CD19 CAR T cells for relapsed B cell malignancies: a phase I dose escalation and expansion trial. Nature Medicine 26:1569-1575 (2020). PMID: 33020647.
- Ortiz-Maldonado V, Frigola G, Espanol-Rego M, et al. Results of ARI-0001 CASRT19 Cells in patients with chronic lymphocytic leukemia and Richter’s transformation. Frontiers in Oncology 12:828471 (2022). PMID: 35174095.
- Medicines and Healthcare Products Regulatory Agnecy, United Kingdom. Consultation on Point of Care Manufacturing (2021). https://www.gov.uk/government/consultations/point-of-care-consultation/consultation-on-point-of-care-manufacturing.
- Ghassemi S, Durgin JS, Nunez-Cruz S, et al. Rapid manufacturing of non-activated potent CAR T cells. Nature Biomedical Engineering 6:118-128 (2022). PMID: 35190680.
- Rajawat YS, Humbert O, Cook SM, et al. In Vivo gene therapy for canine SCID-X1 using Cocal-pseudotyped lentiviral vector. Human Gene Therapy 32:113-127 (2021). PMID: 32741228.
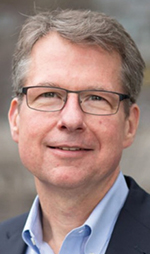
Dr. Rimas Orentas is the Co-founder and Scientific Director of Caring Cross. He earned his PhD from The Johns Hopkins University School of Medicine. After 12 years in academia, he worked for 5 years at the Pediatric Oncology Branch of the NCI, and for a total of 5 years at Lentigen Corporation, where he served as Scientific Director. He currently is an investigator at the Ben Towne Center for Childhood Cancer Research, Seattle Children’s Research Institute, Director for Scientific Integration at CureWorks, and Professor of Pediatrics and Laboratory Medicine and Pathology at the University of Washington School of Medicine.
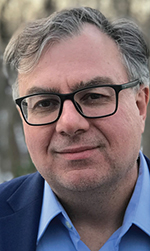
Dr. Boro Dropulić is the Co-founder and Executive Director of Caring Cross, an organization focused on improving business models to support the affordability and accessibility of gene therapy products. Dr. Dropulić earned his PhD from the University of Western Australia and his MBA from the Johns Hopkins University (JHU). He has been in the gene therapy field since the late 1980s.
Total Page Views: 5173