Issue:May 2022
FREEZE-DRYING MICROSCOPY - Unravelling the Complexities of Freeze-Drying Pharmaceuticals With Advanced Microscopy Techniques
INTRODUCTION
Degradation of stored material, either through autolysis or the growth of spoilage organisms, is primarily dependent on the presence of water. Products that are prone to degradation, such as food and pharmaceuticals, must be stabilized by immobilizing or reducing of the water content. For example, vaccines and other biological materials can be stabilized by chilling or freezing, but transporting samples in a frozen state is expensive, and breakdown of freezers may result in the complete loss of valuable product. Alternatively, water can be removed from labile products through air-drying using high processing temperatures, but this can alter the product’s physical and chemical properties and is therefore unsuitable for pharmaceuticals.1
Freeze-drying (lyophilization) removes most of the water in a sample under low temperature and vacuum conditions, providing a dry, active, shelf-stable, and readily soluble product. It is therefore a widely used technique in the manufacture of protein biomolecules for therapeutic and diagnostic applications.2 As well as stabilizing drug products for transport and preventing degradation, freeze-drying reduces the volume of the sample, thus it requires less storage space and can be stored under ambient conditions.
Lyophilized drugs are representing an increasing proportion of the market, with the upward trend particularly strong in the biologics segment, where innovation and drug development is advancing rapidly. In addition, the complexity and strict storage conditions needed to preserve the biological drug activity make freeze-drying a popular formulation technique.
Although used by laboratories since the 1880s and upscaled to industrial scale in the 1930s with commercially available systems, freeze-drying is an incredibly complex process, and research is ongoing to better understand and optimize the process. The three main stages of the freeze-drying cycle (freezing, primary drying, and secondary drying) are often altered and adapted to specific properties of a drug product, and adjustments may be required when scaling up from research and development (R&D) to production. In addition, freeze-drying is a notoriously lengthy process, so pharma and biotech companies can decrease costs by optimizing freeze-drying protocols to speed up timelines and increase product yields.
DIGGING DEEPER INTO LYOPHILIZATION
Being able to directly visualize the freeze-drying process is essential in minimizing the time and cost it takes to develop a protocol for drug development and manufacture. Freeze-drying microscopy (FDM), first developed in the 1960s, combines light microscopy techniques, such as phase contrast and polarized light, with a thermal stage that can accurately control temperature and pressure.
FDM has since become a widely used method that can uniquely determine how a drug product will react to different thermal conditions. Of particular interest are the temperature and pressure required for each of the freezing and drying stages. One of the critical parameters is the collapse temperature (Tc) – the temperature at which the structure of the formulated product weakens and is no longer able to support itself – which can only be reliably measured using FDM. FDM can also be used to determine the eutectic temperature (Teu) – the lowest possible melting temperature over all of the mixing ratios for the involved component species in a mixture of substances – and the potential formation of a barrier due to formation of a product/matrix “skin.”
Researchers at the UK’s National Institute for Biological Standards and Control (NIBSC), led by Dr. Paul Matejtschuk, are using the latest FDM technology to investigate the development of formulation and freeze-drying processes, with a focus on protein therapeutics. The group uses FDM to establish how different formulations affect the freeze-drying process; for example, how drug manufacturers can overcome the challenges of freeze-drying from organic solvents, or stabilizing drug delivery systems such as liposomes.
OPTIMIZING FREEZE-DRIED LIPOSOMAL FORMULATIONS
Liposomal delivery systems are increasingly used in the pharmaceutical industry due to their versatility and their unique ability to entrap both lipophilic and hydrophilic compounds. There are four main types of liposomal delivery platforms that offer varying degrees of stability and specificity (Figure 1). Although there are many liposomal drug formulations currently in clinical trials and several on the market, their high cost and challenges surrounding physical and chemical stability have limited their widespread commercial development.
Liposomes are prone to oxidation or hydrolysis caused by chemical and physical degradation, drug leakage, formation of aggregates, and fusion.4,5 These changes can alter a drug’s formulation properties, affecting not only the product’s shelf-life, but also the in-vivo bio-distribution and pharmacokinetic properties of the drug formulation.6-8
Although aqueous liposomal formations are available on the market, their susceptibility to chemical and physical degradation, as well as the cost of cold-chain distribution, mean that other methods of stabilization are often required. Freeze-drying is therefore a popular and widely studied technique for stabilizing liposomal delivery systems.
The team at NIBSC and Strathclyde recently investigated the liposomal freeze-drying process to improve its reproducibility and compatibility with the high-throughput screening of liposomes. Specifically, the study aimed to identify the formulation and process parameters ideal for the freeze-drying of empty and protein loaded liposomes using Ovalbumin (OVA).9 The group also considered the formulation parameters with regard to presence of protein, lipid selection, lipid charge (neutral, cationic, and anionic), lipid concentration, and the final concentration of the cryoprotectant sucrose, as all of these factors are known to influence freeze-drying. Formulations were freeze-dried in both 96 well plates and vials in parallel.
FDM was used to predict the ideal freeze-drying conditions for liposome-cryoprotectant mixtures, by enabling an estimation of the freezing, collapse, and melt temperatures using a cryostage (FDCS196, Linkam Scientific Instruments) mounted on an optical microscope (BX51, Olympus) connected to a control unit and Liquid Nitrogen Pump (T94 and LNP, Linkam Scientific Instruments).
The samples were frozen using liquid nitrogen at a rate of 10°C/ min until -50°C was reached, after which the temperature was held for 2 minutes before drying was started by applying a 0.1mBar vacuum. To establish the collapse and melt of the samples, the temperature was then ramped up to 20°C at a controlled rate. Images were taken every 20 seconds for the duration of the cycle and physical changes to the liposomal formulations could be observed in real-time (Figure 3).
Results showed the presence of protein adds stability to both neutral and charged formulations, with the same amount of OVA retained after freeze drying (Table 1). The minimal to no leakage of the OVA suggests that pre-cooling the shelf and rapid freezing could prevent egress resulting from the formation of large crystals. In contrast, the liposomal size changed upon rehydration, with cationic liposomes showing the greatest increase in size.
The study demonstrated the ability to freeze-dry liposomal formulations in microplates, as well as vials for the rapid screening, preservation, and optimization of liposomal formulations. Liposomal physicochemical characteristics were preserved regardless of the formulation type, with no loss of protein observed. This positions the freeze-drying process outlined in this experiment as a feasible, transferable, quantifiable, and rapid method for screening and improving the longevity potential of pharmaceutical liposomal products.
OPTIMIZING TOMORROW’S DRUGS
As the biologics market continues to grow, the demand for lyophilized formulations is set to increase. Biopharma companies, when scaling up freeze-drying processes from the development stage, require methods to examine each stage and optimize the speed and cost of the process. The availability of accurate FDM methods is therefore vital for the in-depth investigation of freeze-drying processes and structures. Freeze-drying proteins presents unique challenges due to the need to preserve their biological activity, necessitating equipment that can measure parameters, such as Tc and Te, to ensure the correct temperatures are adhered to.
FDM is being used to analyze the most challenging formulations, such as liposome drug delivery systems, that require sophisticated stabilization methods. By altering and adapting the three main freeze-drying stages to specific formulations, researchers at NIBSC are providing the tools needed for the pharma industry to improve its drug development processes and maintain drug stability when scaling up to production.
REFERENCES
- Ward KR and Matejtschuk P eds. (2019) Lyophilization of Pharmaceuticals and Biologicals – New Technologies and approaches. Methods in Pharmacology and Toxicology, Humana Press.
- Ward KR and Matejtschuk P (2021) Principles of Freeze Drying & Application of Analytical Technologies. In Wolkers W and Oldnehof H (4th ed) Cryopreservation & Freeze Drying Protocols. Methods in Molecular Biology 2180, Humana Press, p99-128.
- Sercombe L, et al. (2015) Advances and Challenges of Liposome Assisted Drug Delivery. Front. Pharmacol. 6:286.
- Zuidam NJ, et al. (1995) Physical (in) stability of liposomes upon chemical hydrolysis: the role of lysophospholipids and fatty acids. Biochimica et Biophysica Acta (BBA)- Biomembranes, 1240(1): 101-110.
- Grit M and Crommelin DJ. (1993) Chemical stability of liposomes: implications for their physical stability. Chemistry and Physics of Lipids. 64(1-3): 3-18.
- Torchilin VP. (2005) Recent advances with liposomes as pharmaceutical carriers. Nature Reviews Drug Discovery. 4(2):145.
- Fransen GJ, Salemink PJM, and Crommelin DJ. (1986) Critical parameters in freezing of liposomes. International Journal of Pharmaceutics. 33(1):27-35.
- Meunier F, Prentice H, and Ringden O. (1991) Liposomal amphotericin B (AmBisome): safety data from a phase II/III clinical trial. Journal of Antimicrobial Chemotherapy. 28(suppl B):83-91.
- Hussain MT, Forbes N, Perrie Y, Malik KP, Duru C, Matejtschuk P. (2020) Freeze-drying cycle optimization for the rapid preservation of protein-loaded liposomal formulations. International Journal of Pharmaceutics 573 (2020)118722. https://doi.org/10.1016/j.ijpharm.2019.118722.
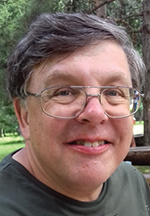
Dr. Paul Matejtschuk leads a team in the development of formulation and freeze- drying processes for the WHO International Standards and other reference materials produced by NIBSC, a centre of the Medicines & Healthcare products Regulatory Agency. He has over 30 years post-doctoral experience across downstream processing, including lyophilization. His most recent focus has been in the biological application of thermal analysis, formulation, and lyophilization of biologicals, high throughput screening methods, application of Design of Experiments (DoE) and Process Analytical Technology (PAT) in freeze-drying as well as the measurement of residual water and its impact on the stability of biologics.
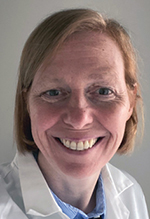
Prof. Yvonne Perrie is Professor in Drug Delivery within the Strathclyde Institute of Pharmacy and Biomedical Sciences, University of Strathclyde, Glasgow, Scotland. Her research is multi-disciplinary and is focused on the development of drug delivery systems to facilitate the delivery of drugs and vaccines, thus providing practical solutions for current healthcare problems. She is an internationally recognized expert in the field of liposomes and particulate drug delivery research with a strong track-record of high impact publications, with approximately 130 peer-reviewed manuscripts plus 5 textbooks and 6 patents.
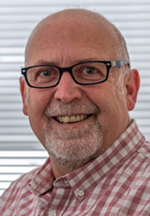
Dr. Duncan Stacey is Sales and Marketing Director at Linkam Scientific Instruments. He is focused on the development of new markets and strategic OEM partners and he brings a wealth of technical and commercial experience to Linkam. Before joining Linkam, he worked in Sales, Marketing, and Product Development for some of the leading photonics companies in imaging, spectroscopy, and microscopy. He earned his PhD in 1993 from University of Liverpool in Optics and Spectroscopy.
Total Page Views: 10259