Issue:March 2024
FORMULATION FORUM - Nanoparticle Technologies Used in Ocular Drug Delivery
INTRODUCTION
As we continue to discover new and innovative small molecules and therapeutic compounds, over 80% of those candidates in the drug pipeline are challenging to develop due to their poor solubility and bioavailability.1 That has created a roadblock for bringing in novel drugs to the market across all modalities. Though there are lots of publications and knowledge accumulation in oral and parenteral routes of drug delivery, the topical route of administration, more specifically, ocular delivery has been subject of continued interest in development of innovative drugs due, in part, to poor bioavailability (ca. 5%), and lack of safety and regulatory challenges.2 The latter requires a thorough understanding of nanotechnologies applicable to innovative formulations for efficient delivery to target tissues in the eyes for treating diseases of the anterior segment.3
Although many drugs are available for the treatment most of eye diseases, there are still many ocular barriers, such as tear film, corneal, conjunctival, and blood barriers, that cause bioavailability issues.4 Thus, finding ways to deliver drugs to the specific tissue in front and back of the eyes could be challenging because of a lack of drug solubility and permeation through the corneal membrane or in the interior eyes. Otherwise, drug delivery may require implants through invasive surgery. Currently, most eye drugs still focus on delivery through the topical self-administrative route, and the nanotechnology-based drug delivery system (NODS) remains the acceptable and effective way for topical administration of drugs.5 NODS is applicable to those entities based on lipid nanoparticles, such as micelles, liposomes, cubosomes, nano- and microemulsions, dendrimers, polymeric hydrogels, among others.6
The following will describe the basic understanding of eye diseases, challenges in ocular drug delivery, and the future trends in development of innovative drugs.
OCULAR DISEASES
Eye diseases affect over 2.2 billion people globally according to the World Health Organization (WHO), and a majority of these diseases are preventable and treatable. Among the most common and debilitating eye diseases are bacterial and fungal infections, cataracts, age-related macular degenerative (AMD), glaucoma, conjunctivitis, diabetes retinopathy, retinoblastoma, among others. The root cause of some of these diseases however remains elusive.7 Fungal keratitis, for example, occurs in traumatic cornea and is caused by fungus, such as Candida albicans, Candida glabrata, and others. Diabetes retinopathy results in loss of vision by leakage of blood from the back of the eyes. It is caused by oxidative stress and inflammation upregulated by proinflammatory mediators. Glaucoma is related to increased intraocular pressure, whereas AMD occurs due to irregular angiogenesis in the retinal epithelial that leads to vision loss and blindness if not treated on time.
OCULAR DELIVERY
Figure 1 shows the route of administration of drug in the eyes. These include topical, subconjunctival injection, intravitreal injection, retrobulbar injection, and intracameral injection. The topical route, however, is non-invasive, widely used, and remains a self-administrative route. The challenges involve the immediate clearance of drugs by spillage and tear drainage that leads to reduced bioavailability.
The topical route of administration is a preferred method for treatment of many ophthalmic diseases. It allows only a limited amount of drug to permeate through the precorneal surface. It helps cross permeation of drugs through the cornea to aqueous by passive transcellular pathway. It is however limited by the membrane tight junctions that allow only 5% of drug absorption.8 Therefore, solubility, molecular weight, ionic charges, lipophilicity, and pKa are all important properties that enable permeation, absorption, and bioavailability enhancement of drugs. Figure 2 illustrates the topical route of administration and leading pathways for absorption and clearances of drugs.
As indicate in Figure 2, the drug is distributed from the precorneal surface to the anterior segment through the cornea and conjunctiva/sclera pathways, several barrier layers, and eventually leading to systematic circulation and elimination. This is a complex mechanism, but drug distribution via nanoparticles through these barriers depends upon the molecular weight, lipophilicity, and ocular transporters including proteins and melanin binding.9
NANOTECHNOLOGIES FOR OCULAR DELIVERY
Nanoparticles are derived from a range of excipients and polymers suited for encapsulating and delivering drugs to the target tissues. It is important to understand the physico-chemical properties of these excipients and their role played in designing, formulating, loading and carrying, and protecting of drugs.
Range of Excipients/Polymers Include
Lipids: DSPC, DPPC, DMPC, DOPC, DPPE, Soy lipids, DSPE-mPEG 2000; Cationic lipids (DOTAP), among others
Oils/Glycerides/Surfactants: Mono-, di- and triglycerides, fatty acids; castor oil, polyoxyl 40 hydrogenated castor oil, polyoxyl 35 castor oil, polyoxyl 15 hydroxystearate, polysorbate 80, vitamin E-TPGS, Poloxamer 407, Poloxamer 188, Transcutol, among others
Polymers: PLGA, PCL, PVA, HPMC, HPC, povidone, copovidone, Soluplus, carboxymethyl sodium, chitosan, hyaluronic acid, alginate, gellan gum, among others
Lipid Nanoparticles as Vehicles for Ocular Delivery
Saturated lipid nanoparticles, nanostructured lipid carriers, liposomes, cubosomes, micro- and nanoemulsions; nanosuspensions, dendrimers, micelles, among others are a range of carriers for ocular or ophthalmic delivery. These assemblies ranging in size between 10 nm and 1000 nm are widely utilized for delivery of a range of therapeutics to overcome the physiological barriers and to target specific ocular tissues. As stated earlier, these are derived from a range of pharmaceutically accepted and listed FDA inactive ingredients, including excipients, polymers, and co-solvents and surfactants. Thus, for designing appropriate safe and efficacious formulations by selecting one or more ingredients, the list of approved ingredients further facilitates the regulatory process. For example, hyaluronic acid, alginate, and/or cellulosic approved excipients are commonly preferred for enhancing the residence time in the cornea and conjunctiva.10 Tatke et al investigated triamcinolone nanoparticles composed of glyceryl monostearate and Compritol 888 to enhance the trans-corneal permeation of drug.11 Kalam, et al evaluated the solubility and ocular bioavailability of tedizolid phosphate nanocrystals using stearyl amine and benzalkonium chloride and found the drug was stable over 180 days at 25°C and 37°C and showed extended release over 12 hours.12 Liu, et al evaluated hydrophilic moxifloxacin encapsulated lipid-polymer nanoparticles containing egg phospholipid, DSPE, cholesterol, and chitosan, which was surface modified with hyaluronic acid for targeting. It was observed it improved permeation and bioavailability.13 Ren, et al used cholesterol hemisuccinate with medium chain triglycerides to improve the solubility, loading, and stability of azithromycin in the liposomes.14 In a study demonstrating the use of SLN, NLC, and eye drop formulations of brimodinine, El Salamouni, et al observed NLC enhanced 1.3-fold higher permeability compared to SLN due to stronger affinity with cell membrane, leading to sustained release and greater impact on lowering the intraocular pressure compared to SLP and eye drops.15 Morsi, et al investigated acetazolamide nanoemulsions composed of polysorbate 80, polyxyl 35 castor oil, Transcutol, and peanut oil in combination with xanthan gum, HPMC, or Carbopol gel and found stability and efficacy of drug were improved compared to eye drops and tablets.16 Table 1 cites a few examples of NPs in ocular delivery.
CHARACTERIZATION OF NANOPARTICLES
Nanoparticles for ocular delivery, like any other applications, require a thorough characterization due in part to particle size uniformity, polydispersity, and homogeneity of the formulations. The visual appearance for identifying the insoluble particulates, transparency vs. translucency, or milky white are important critical quality attributes and must be optimized.31
Monitoring the morphological changes, stability, zeta potential, surface tension, refractive index, and rheological properties are equally important for maintaining the product quality. For instance, a zeta potential around ±20 mV is appropriate for electrostatic attachment with the corneal surface, and higher values between ±20-40 mV is also desired for preventing aggregation and maintaining the stability of these particulates. Citing a cubosomal formulation with zeta potential of -30.2 mV, for instance, it improved the bioavailability and activity as compared to Alphagan® eye drops.32
Dynamic light scattering (DLS) is typically used to characterize the particle size and polydispersity index (PDI) using a Malvern or Coulter counter analyzer. While PDI closer to 0 indicates a unimodal distribution, the values closer to 1 indicate a heterogeneous system. For ocular delivery, smaller PS and PDI particles are preferred to enhance the corneal permeation and bioavailability. Ocular formulations with low viscosity ranging between 2 mPas and 3 mPas allows better patient compliance; however, the higher viscosity allows for sustained release, less frequent dosing, and enhanced bioavailability.31
Surface tension of ocular formulations is critical for patient compliance and retention of drug to the corneal surface. An ocular dose is typically 5-15 mL with few exceptions for commercial eye drops. Ocular formulations with surface tension of < 35 mN/m can lead to discomfort in the eye, but with surface tensions of 40-50 mN/m, they can provide more relief and comfort to the eye.33 Likewise, pH and osmolality ranging between 4 and 8 and 231 and 446 mOsm/kg, respectively, can lead to improved stability and significant permeation of drug through the eye.7
FDA APPROVED DRUGS
As nanoparticle technologies continue to address the challenges with poorly soluble molecules across all the dosages with the aim to increase solubility and enhance bioavailability, the industry is weighing options for identifying and adapting new excipient technologies for bringing innovative drugs faster to market. That has driven the sense of urgency for finding technologies for unmet medial needs in ocular formulations. Consequently, several drugs have been approved employing nanoparticle technologies for the treatment of diseases affecting the anterior segment of the eye. Table 2 lists a number of approved ocular drugs to market over the years.
SUMMARY & FUTURE PERSPECTIVES
Ocular drug delivery is challenging due to many anatomical barriers. Nanoparticle technologies in ocular delivery, however, have demonstrated wide utilities across all molecules, small and large. As shown in Table 2, several of the approved drugs and many more molecules are undergoing clinical development. Citing a few examples, NCT03001466 is undergoing Phase 2 and is composed of urea loaded in Poloxamer 407 nanoparticles for dry eye syndrome, while NCT02420834 is composed of a liposomal spray used for artificial tears for dry eye. Clinical trial (NCT02845674) involving Cyclosporine A is undergoing Phase 3 as a 0.09% micellar solution for dry eye syndrome.34 With the acceptance of nanotechnologies in the recent past, we believe nanoparticle technologies will pick up the steam in ocular delivery for the launch of new molecules to market with more innovative polymeric and lipid assemblies like cubosomes.35,36
Ascendia Pharma with its enabling platform technologies, NanoSol® (nanosuspension), EmulSol® (nanoemulsion) and LipidSol® (lipid nanoparticles) are primed to address the challenges with poorly soluble drugs. For example, using EmulSol® technology, Ascendia can address the particle size with its top down/or bottom-up approach with FDA-approved excipients in nanoparticle formulations composed of 0.05% Cyclosporine A with a particle size <50 nm and composed of soy bean oil, lecithin, and polysorbate 80.37
With its cGMP sterile manufacturing capabilities, Ascendia is poised to tackle the challenges of formulation development and manufacturing of poorly soluble molecules to address the unmet medical needs to design better and smarter nanoparticle ocular formulations in the future.
REFERENCES
- J. Huang and S. Ali, Lipid Nanoparticles: Tackling solubility challenges with lipid-based technologies for oral and injectable formulations, Drug Dev. Delivery, 2022 November.
- J. Zhang, J. Jiao, M. Niu, X. Gao, G. Zhang, H. Yu, X. Yang and L. Liu, Ten years of knowledge of nano-carrier based drug delivery systems in ophthalmology: Current evidence, challenges, and future prospectives, Int. J. Nanomedicine, 2012, 16, 6497-6530.
- S. Li, L. Chen and Y. Fu, Nanotechnology-based ocular drug delivery systems: Recent advances and future prospects, J. Nanotechnology, 2023, 21, 232.
- I. Elsayed and S. Syed, Tailored nanostructured platforms for boosting transcorneal permeation: Box–Behnken statistical optimization, comprehensive in vitro, ex vivo and in vivo characterization, Nanomedicine 2017, 12, 7947-7962.
- A. L. Onugwa, C. S. Nwagwu, O. S. Onugwu, A. C. Echezona, C. P. Agbo, S. A. Ihim, P. Emeh, P. O. Nnamani, A. A. Attama, and V. V. Khutoryanskiy, Nanotechnology based drug delivery systems for the treatment of anterior segment eyes diseases, J. Control. Release, 2023, 354, 465-468.
- C. R. Lynch, P. P. D. Kondiah, Y. E. Choonara, L. C. du Toit, N. Ally and V. Pillay, Hydrogel biomaterials for application in ocular drug delivery, Frontiers Bioeng. Biotech., 2020, 8, 228.
7. S. Ahmed, M. M. Amin and S. Sayed, Ocular drug delivery: A comprehensive review, AAPS PharmSciTech, 2023, 24, 66. - P. L. Destruel, N. Zeng, M. Maury, N. Mignet and V. Boudy, In vitro and in vivo evaluation of in situ gelling systems for sustained topical ophthalmic delivery: state of the art and beyond, Drug Discovery Today, 2017, 22, 638–651.
- S. Gorantla, V. K. Rapalli, T. Waghule, P. P. Singh, S. K. Dubey, P. N. Saha, and G. Sanghavi, Nanocarriers for ocular drug delivery: current status and translational opportunity, RSC Adv. 2020, 10, 27835-27855.
- E. Sanchez-Lopez, M. Espina, S. Doktrovova, E. B. Souto and M. I. Garcia, Lipid nanoparticles (SLN, NLC): Overrcoming the anatomical and physiological barriers of the eye- Part I- Barriers and determining factors in ocular delivery, Eur. J. Pharm. Biopharm., 2017, 110, 70-75.
- A. Tatke, N. Dudhipala, K. Yadav Janga, S. P. Balguri, B. Avula, M. M. Jablonski, and S. Majumdar, In Situ Gel of Triamcinolone Acetonide-Loaded Solid Lipid Nanoparticles for Improved Topical Ocular Delivery: Tear Kinetics and Ocular Disposition Studies, Nanomaterials, 2018, 9, 33.
- M.A. Kalam, M. Iqbal, A. Alshememry, M. Alkholief, and A. Alshamsan, Fabrication and characterization of tedizolid phosphate nanocrystals for topical ocular application: Improved solubilization and in vitro drug release, Pharmaceutics, 2022, 14, 1328.
- Liu, Y. Lian, Q. Fang, L. Liu, J. Zhang, J. Li, Hyaluronic-acid-modified lipid-polymer hybrid nanoparticles as an efficient ocular delivery platform for moxifloxacin hydrochloride, Int. J. Biol. Macromol. 2018, 116, 1026–1036.
- T. Ren, X. Lin, Q. Zhang, D. You, X. Liu, X. Tao, J. Gou, Y. Zhang, T. Yin, H. He, and X. Tang, Encapsulation of azithromycin ion pair in liposome for enhancing ocular delivery and therapeutic efficacy on dry eye, Mol. Pharm., 2018, 15, 4862–4871.
- N. S. El Salamouni, R. M. Farid, A. H. El-Kamel and S. S. El-Gamal, Effect of sterilization on the physical stability of brimonidine-loaded solid lipid nanoparticles and nanostructured lipid carriers, J. Microencapsulation, 2018, 35, 102–113.
- N. Morsi, M. Ibrahim, H. Refai and H. El Sorogy, Nanoemulsion-based electrolyte triggered in situ gel for ocular delivery of acetazolamide, Eur. J. Pharm. Sci., 2017, 104, 302–314.
- E. Terreni, E. Zucchetti, S. Tampucci, S. Burgalassi, D. Monti, P. Chetoni, Combination of Nanomicellar Technology and In Situ Gelling Polymer as Ocular Drug Delivery System (ODDS) for Cyclosporine-A, Pharmaceutics, 2021, 13, 192.
- Y. Yu, D. Chen, Y. Li, W. Yang, J. Tu, and Y. Shen, Improving the topical ocular pharmacokinetics of lyophilized cyclosporine A-loaded micelles: formulation, in vitro and in vivo studies, Drug Deliv. 25 (2018) 888–899.
- S. Akhter, M. Anwar, M. A. Siddiqui, I. Ahmad, J. Ahmad, and M. Z. Ahmad, A. Bhatnagar, and F. J. Ahmad, Improving the topical ocular pharmacokinetics of an immunosuppressant agent with mucoadhesive nanoemulsions: Formulation development, in-vitro and in-vivo studies, Colloids Surf. B: Biointerfaces, 2016, 148,19-29.
- A. L. Onugwu, A. A. Attama, P. O. Nnamani, S. O. Onugwu, E. B. Onuigbo, and V. V. Khutoryanskiy, Development and optimization of solid lipid nanoparticles coated with chitosan and poly(2-ethyl-2-oxazoline) for ocular drug delivery of ciprofloxacin, J. Drug Deliv. Sci. Technol., 2022, 74, 103527.
- W.S. Alharbi and K.M. Hosny, Development and optimization of ocular in situ gels loaded with ciprofloxacin cubic liquid crystalline nanoparticles, J. Drug Deliv. Sci. Technol., 2020, 57, 101710.
- I.A. Khalil, I.H. Ali, I.M. El-Sherbiny, Noninvasive biodegradable nanoparticles-in nanofibers single-dose ocular insert: in vitro, ex vivo and in vivo evaluation, Nanomedicine, 2019, 14, 33–55.
- W. Zeng, Q. Li, T. Wan, C. Liu, W. Pan, Z. Wu, G. Zhang, J. Pan, M. Qin, Y. Lin, C. Wu, Y. Xu, Hyaluronic acid-coated niosomes facilitate tacrolimus ocular delivery: Mucoadhesion, precorneal retention, aqueous humor pharmacokinetics, and transcorneal permeability, Colloids Surf. B: Biointerfaces, 2016, 141, 28–35.
- D. Modi, M.H. Mohammad, V. Warsi, M. Garg, P. Bhatia, and G.K. K. Jain, Formulation development, optimization, and in vitro assessment of thermoresponsive ophthalmic pluronic F127-chitosan in situ tacrolimus gel, J. Biomater. Sci. Polym. Ed., 2021, 32, 1678–1702.
- B. Xu and T. Liu, Travoprost loaded microemulsion soaked contact lenses: Improved drug uptake, release kinetics and physical properties, J. Drug Deliv. Sci. Technol., 2020, 57, 101792.
- M. Gomez-Ballesteros, J. J. Lopez-Cano, I. Bravo-Osuna, R. Herrero-Vanrell, and I. T. Molina-Martinez, Osmoprotectants in hybrid liposome/HPMC systems as potential glaucoma treatment, Polymers 2019, 11, 929.
- N. Seedat, R.S. Kalhapure, C. Mocktar, S. Vepuri, M. Jadhav, M. Soliman, T. Govender, Co-encapsulation of multi-lipids and polymers enhances the performance of vancomycin in lipid–polymer hybrid nanoparticles: In vitro and in silico studies, Mater. Sci. Eng., 2016, C 61, 616–630.
- S. Kumari, M. Dandamudi, S. Rani, E. Behaeghel, G. Behl, D. Kent, N.J. O’Reilly, O. O’Donovan, P. McLoughlin, and L. Fitzhenry, Dexamethasone-Loaded Nanostructured Lipid Carriers for the Treatment of Dry Eye Disease, Pharmaceutics, 2021, 13, 905.
- G. Tan, J. Li, Y. Song, Y. Yu, D. Liu, and W. Pan, Phenylboronic acid-tethered chondroitin sulfate-based mucoadhesive nanostructured lipid carriers for the treatment of dry eye syndrome, Acta Biomater., 2019, 99, 350–362.
- T. Fu, J. Yi, S. Lv, and B. Zhang, Ocular amphotericin B delivery by chitosan-modified nanostructured lipid carriers for fungal keratitis-targeted therapy, J. Liposome Res., 2017, 27, 228–233.
- M. Singh, S. Bharadwaj, K. E. Lee, and S. G. Kang, Therapeutic nanoemulsions in ophthalmic drug administration: concept in formulations and characterization techniques for ocular drug delivery. J Control Release. 2020, 328, 895-916.
- A. E. Eldeeb, S. Salah and M. Ghorab, Formulation and evaluation of cubosomes drug delivery system for treatment of glaucoma: ex-vivo permeation and in-vivo pharmacodynamic study, J Drug Deliv Sci Technol. 2019, 52, 236–47.
- M. H. Grgurevic, M. Juretic, A. Hafner, J. Lovric, and I. Pepic, Tear fluid-eye drops compatibility assessment using surface tension. Drug Dev. Ind. Pharm. 2017, 43, 275–282.
- Clinical Trials: https://classic.clinicaltrials.gov/ ct2/home.
- H. E. Teba, I. A. Khalil, and H. M. El Sorogy, Novel cubosomes based systems for ocular delivery of acetazolamide, Drug Delivery, 2021, 28, 2177-2186.
- N. Mehra, M. Aqil and Y. Sultana, A grafted copolymer-based nanomicelles for topical ocular delivery of everolimus: Formulation, characterization, ex-vivo permeation, in-vitro ocular toxicity, and stability study. Eur. J. Pharm. Sci., 2021, 159, 105735.
- J. Huang, Compositions for nano-emulsion delivery systems, US Patent 10,251,837 (2019)
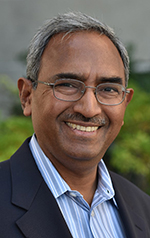
Shauket Ali, PhD
Sr. Director, Scientific Affairs & Technical Marketing
Ascendia Pharmaceuticals
shaukat.ali@ascendiapharma.com
www.ascendiapharma.com
Dr. Shaukat Ali joins Ascendia Pharmaceuticals Inc. as Senior Director of Scientific Affairs and Technical Marketing after having worked in the pharma industry for many years. His areas of expertise include lipid chemistry, liposomes, lipid nanoparticles, surfactant-based drug delivery systems, SEDDS/SMEDDS, oral and parenteral, topical and transdermal drug delivery, immediate- and controlled-release formulations. He earned his PhD in Organic Chemistry from the City University of New York and carried out his post-doctoral research in Physical Biochemistry at the University of Minnesota and Cornell University. He has published extensively in scientific journals and is inventor/co-inventor of several US and European patents.
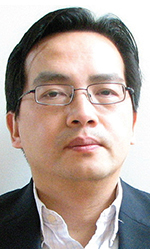
Jim Huang, PhD
Founder & CEO
Ascendia Pharmaceuticals
j.huang@ascendiapharma.com
www.ascendiapharma.com
Dr. Jim Huang is the Founder and CEO of Ascendia Pharmaceuticals, Inc. he earned his PhD in Pharmaceutics from the University of the Sciences in Philadelphia (formerly Philadelphia College of Pharmacy and Sciences) under Joseph B. Schwartz. He has more than 20 years of pharmaceutical experience in preclinical and clinical formulation development, manufacturing, and commercialization of oral and parenteral dosage forms. His research interests are centered on solubility/bioavailability improvement and controlled delivery of poorly water-soluble drugs through nano-based technologies.
Total Page Views: 4014