Issue:October 2024
FORMULATION FORUM - Lyophilization Technology - An Enabler for Stable Formulations of Small & Large Molecules
INTRODUCTION
As more new chemical entities continue to be discovered, over 80% of molecules are poorly soluble and less bioavailable, many of which are unstable in aqueous formulations. Thus, developing a liquid dosage might be an impediment for long-term storage and shelf-life. This is due, in part, to inherent physical and/or chemical instability stemmed from morphological changes, such as aggregation, pH changes or hydrolysis, isomerization (enantiomers and diastereomers), and/or functional group rearrangement, which could lead to change in potency and adverse effects of drug molecules.1 An instability constitutes a barrier in commercial development and manufacturing of pharmaceutical products. Thus, efforts continue to identify the appropriate technologies and excipients to stabilize the drugs in solid state to overcome their conversion from one form to others and to minimize generation of new impurities and enhance shelf-life.2 Thus, lyophilization or freeze-drying technology is one of the ideal methods that leads to immobilization of drug and other components that remain in solid or powder state for extended periods without concerns of degradation. In addition, lyophilization has also been applied to improve drug solubility by means of amorphous solid dispersions.
Over the years, many drugs have been approved as lyophilized products to improve shelf-life, ease of handling, and enhanced stability. Table 1 shows the top 10 approved blockbuster drugs marketed in lyophilized forms.3
These drugs, when lyophilized, are stable and completely eliminate the need for cold storage or subzero temperatures, making storage and transportation much more efficient. For many of the molecules inherently insoluble, lyophilization improves their solubility, making them easier to store and administer when warranted, and also potentially increases their efficacies. The key requirement for sterile lyophilization is the facility that complies with an ISO 5 (Class 100) environment equipped with Clean-In-Place (CIP) and Steam-In-Place (SIP) capabilities to support sterile and aseptic processes. Extensive validation and monitoring of cleaning procedures and sterile conditions is also required in lyophilization operation.
LYOPHILIZATION PROCESS
The lyophilization process can be divided into three steps. The first step is freezing leading to solidification. The second step is primary drying under cold or freezing conditions leading to ice sublimation. The third step is the secondary drying conditions leading to removal of unfrozen water/solvents (Figure 1), and Table 2 shows the evolution of process parameters and corresponding states formed during successive stages of lyophilization.4 Freezing and drying times, temperature, and chamber pressure all should be adjusted as deemed necessary depending upon product composition, structure, and volume.
Thermophysical properties, such as eutectic temperature (Teu), glass transition temperature of cryo-concentrated solute (Tg’), collapse temperature (Tc) of lyophilized product, and glass transition temperature of dried product (Tg) are critical for lyophilization cycles and to obtain the quality product. Sublimation is the primary driving force for drying samples in frozen state that facilitates the removal of solvent from the sample. The primary drying remains the longest process for removal of solvent by sublimation, while secondary drying takes 3-10 hours depending upon the sample properties.5 Sublimation of water, for example, takes place at 4.5 mmHg and at 0.009oC at eutectic phase interfacing solid, liquid, and vapor, so-call triple point of phase diagram.6,7 Let’s take a closer look into three processes during the lyophilization process.
Freezing
This process requires the cooling of material in aqueous solution resulting in formation of ice nuclei and crystals. As the freezing process continues, more water molecules continue to nucleate into ice crystals, resulting in increasing the viscosity of solution and solute concentrations. This leads to phase segregation between the crystalline phase of ice crystals and an amorphous solute phase, represented by Tg’. For solutes at which they crystallize, the temperature must be below the eutectic mixture (Teu).8 The unfrozen water left after crystallization at atmospheric pressure remains in liquid state, while freezing continues during lyophilization. The freezing takes place at atmospheric pressure.
Primary Drying
It is a process that involves sublimation of ice from the frozen product. It requires (i) first heat transfers from the shelf to frozen solution, and (ii) ice sublimation starts at reduced pressure as the water vapors relieved through dried portion from upper to bottom level of the vials. The product temperature (Tp) must be controlled and should be kept 2°C to 5°C below the collapse temperature (Tc) or glass transition temperature of solute concentration in the frozen state. The collapse temperature (Tc) can be determined by freeze-drying microscopy (FDM).9 At the end of primary drying, adsorbed water remains within the product and does not get sublimed during the process, but eventually is removed during the secondary drying step that takes place at relatively higher temperature than primary dying.
Secondary Drying
Secondary drying involves the removal of unfrozen water from the product. It is accomplished at reduced pressure and relatively higher shelf-temperature but lower than the glass transition temperature of product (Tg). A higher temperature is necessary to reduce the residual moisture content to a minimal level by isothermal desorption, in which the bound water is desorbed from product. This is important to raise the temperature slowly without damaging the lyophilized product. A rapid rise in temperature may lead to collapse of the amorphous product. Therefore, it is necessary to set the parameters right for each state of the lyophilization cycle, most specifically, the temperature close to freeze-dried temperature of product (Tp). It is a crucial step to control the residual moisture level <1% as the higher moisture may compromise the stability of drug product as it can act as plasticizer.
For storage stability of lyophilized product, the glass transition temperatures of polymers are an important consideration. For example, glass transition temperatures of anhydrous polymers are not necessarily the same as the glass transition temperatures of freeze-dried polymeric nanoparticles. For example, a lower Tg of PLGA is evident in the freeze-dried state compared to pure polymer.10 In presence of moisture, water acts as plasticize leading to lower the Tg of polymer and increasing the polymer chain mobility. All these events could lead to drug precipitation from the amorphous state of lyophilized powder, and likely could lead to degradation of drugs. With PLGA, for instance, in dried power, the addition of 3.7 mg/g of water can lead to lower Tg to 35.3°C from 47.2°C in pure polymeric state.10 Evidently, increasing the humidity could affect the physical state of freeze-dried product by shifting the Tg of the formulation to below the storage temperature, leading to re-crystallization of drug. Any morphological changes in the freeze-dried powder can be easily seen before and after lyophilization. For example, the freeze-dried powder should occupy the same volume as the original frozen mass, suggesting the process is correctly carried out. If it is collapsed during the process, shrinkage and change in the texture and color are highly evident.11 SEM and AFM techniques are also used to further demonstrate the physical state of the lyophilized powder.
The thermophysical properties of the ingredients as summarized in Table 2 will guide the selection of processing parameters for lyophilization cycles to achieve robust powders.1 The lyophilized products of dispersed systems are typically characterized for particle size distribution, zeta potential, polydispersity index for nanoparticles, drug contents, moisture contents, thermal analysis, reconstitution time, microscopic analyses (SEM, TEM), among others.
With development of freeze-dried product of nanoparticles, for instance, lyophilization is ideally suited process for stabilization and maintaining the particle size distributions in a lyophilizate. Other attributes such short reconstitution time, low residual moisture, and drug encapsulation efficiency are important for an optimized drug product. For instance, for freeze drying of fish oil in polycaprolactone (PCL), freezing is carried out at -30, -20 and -10°C, and primary drying takes place at -50°C at 0.05 mbar pressure,12 while for vitamin E in PCL, freezing is carried out at -45°C for 120 min, while primary drying takes place at -20°C for 8 hours, and secondary drying takes about 6 h at +20°C.13 In encapsulation of docetaxel in hyaluronic acid, the freezing takes place at liquid nitrogen, the primary drying requires -35°C for 60 h at 50 mTorr, while the secondary drying requires 0°C for 24 h.14
ROLE OF CRYOPROTECTANTS
Stress during lyophilization could lead to breaking of cakes, leading to destabilization of encapsulated drugs or exposure of drug out of freeze-dried powder. It is important to protect the drugs from undesired consequences by selecting the right excipients, especially in the freezing and primary drying stages of lyophilization cycles. Therefore, cryoprotectants are carefully selected to prevent premature process termination and to give higher yields.
Cryoprotectants are classes of excipients that protect and stabilize the amorphous drug in either encapsulated or free state. The mechanism by which the drugs stabilized are by H-bonding with cryoprotectants with polar groups at the surface of the NPs. In addition, these ingredients preserve the native structures of nanoparticles (NPs) by serving as water substituents. Most of the cryoprotectants are sugars, such as mannitol, trehalose, sucrose, glucose, and lactose among others with their critical process temperatures. Various polymers are also used as stabilizers and protectants. For example, polyvinyl alcohol (PVA) is another excipient used as a cryoprotectant to stabilize the NPs.9 Table 3 shows the a few representative drugs and polymers with respect to sugars as being used as cryoprotectants.
The stability of drug is due to restricted mobility within the glassy cryoprotectant matrix that prevents aggregation and protects against the mechanical stress of ice crystals. The freezing step requires the sample is still frozen at atmospheric pressure and remains solidified during the primary drying process. In a study, mannitol showed phase separation during lyophilization of nanoparticles, but by adding 1% NaCl, it prevents the crystallization of mannitol and maintains the amorphous during freezing state. Beyond their protective nature during the lyophilization, the cryoprotectants also impact the drying stages and could help modify the collapse temperature (Tc) of the product. The higher Tc means a faster sublimation rate in the primary drying process. Table 4 shows the list of commonly used cryoprotectants and their critical physical process temperature used in lyophilization.7
LYOPHILIZATION OF mRNA IN LNPS
Meulewaeter et al investigated the freeze drying of Covid-19 vaccines in LNPs composed of ionizable lipid C12-200, DSPC, cholesterol,Dimyristoyl-rac-glycerol-3-methoxypropylethylene glycol-2000 (DMG-PEG 2000) in composition of 50:10:38.5:1.5 mol%, respectively.15 Precision Nanosystem’s NxGen microfluidic system was used to prepare the LNP suspensions, and upon dialysis with membrane (MWCO 12-14 kD), ethanol was removed, and the LNPs were stored in Tris, PBS, and at pH 7.4. The freeze drying of aqueous suspension of LNPs was carried out with 25% sucrose or trehalose in Tris or PBS buffer composed of 15.6 mg/ml of mRNA and 12.5 m/v% protectant, which is subjected to the freeze-drying process in each 400-μl of protectant and mRNA LNP suspension. The encapsulation efficiency of mRNA/LNPs was determined by Quanti-iT RiboGreen assay. Ai et al have demonstrated the stability of mRNA/LNPs after lyophilization at 4°C and 25°C, but at 42°C, the integrity of mRNA was compromised.16 Muramatsu et al used freeze-drying temperature -45°C (VirTis Genesis Pilot Lyophilizer) with primary drying step at -25°C at 20 mTorr and secondary drying step at 30°C at 20 m Torr, and samples were monitored for their stability at -80°C, -20°C, 4°C, 25°C, and 42°C.17
SUMMARY & FUTURE PERSPECTIVES
With the stability and solubility challenges of small and large molecules, lyophilization remains one of the preferred methods for enhancing the shelf-life and for enabling ambient temperature storage conditions for life-saving medicines that help handling and transporting across the globe. With continued interest in the development of small and large molecules for rare diseases and life-threatening ailments, lyophilization technology remains the most preferred approach for a range of biological and small molecules. With a continued increase in poorly soluble molecules (highly crystalline requiring non-conventional solubilization technology), lyophilization is highly applicable for enhancing the solubility of drugs by converting them in amorphous dispersions. Ascendia’s enabling technologies, including AmorSol®, EmulSol®, NanoSol®, and LipidSo™, are most ideally suited for improving the solubility and bioavailability of drug molecules. For vaccines and large molecules requiring the lyophilization process, LipidSol™ can be used for the development of those drugs and improve the stability over an extended period. Our state-of-the-art cGMP clean room lyophilizer can be used for development of sterile drug products, as shown in Figure 2.
REFERENCES
- G. Degobert and D. Aydin, Lyophilization of nanocapsules: Instability sources, formulation and process parameters, Pharmaceuticcs, 2011, 13, 1112; (b) N. Kumar and U. Nautiyal, A review article on lyophilization techniques used in pharmaceutical manufacturing, Int. J. Pharm. Med. Res., 2017, 5, 478-484.
- H. Kawasaki, T. Shimanouchi and Y. Kimura, Recent development of optimization if lyophilization process, Hindawi J. Chemistry, 2019, article 9502856, 14 pageS.
- Lyophilized marketed drugs: https://particlesciences.com/blog/lyophilization-of-pharmaceuticals-an-overview/.
- D. S. Przic, N. L. J. Ruzic, and S. D. Petrovic, Lyophilization – the process and industrial use, Chem. Ind. 2004, 12, 552-562.
- A. Molnar, T. Lakat,A. Hosszu, B. Szebeni, A. Balogh, L. Orfi, A. J. Sabo, A. Fekete and J. Hordea, Lyophilization and homogenization of biological samples improves reproducibility and reduces standard deviation in molecular biology techniques, Amino Acis, 2021, 53, 917-928.
- K. A. Gaidhani, M. Harwalkar, D. Bhambre, and P. S. Nirgude, Lyophilization/freeze drying : A review, World J. Pharm. Res., 2015, 4, 516-543.
- A. Baheti, L. Kumar, and A. K. Bansal, Excipients used in lyophilization of small molecules, Excipient, J. Excipients and Food Chem. 2010, 1 (1), 41-53.
- W. M. Khattab, E. E. Zein El-Dein, and S. A. El-Gizawy, Formulation of Lyophilized Oily-Core Poly-ε-Caprolactone Nanocapsules to Improve Oral Bioavailability of Olmesartan Medoxomil. Drug Devel. and Ind. Pharm. 2020, 46, 795-805.
- W. Abdelwahed, G. Degobert, and H. Fessi, H. Investigation of Nanocapsules Stabilization by Amorphous Excipients during Freeze Drying and Storage. Eur. J. Pharm. Biopharm. 2006, 63, 87-94.
- M. Holzer, V. Vogel, W. Mäntele, D. Schwartz, W. Haase, and K. Langer, Physico-Chemical Characterization of PLGA Nanoparticles after Freeze-Drying and Storage. Eur. J. Pharm. Biopharm. 2009, 72, 428-437.
- J. T-W. Wang, U. Martino, R. Khan, M. Bazzar, P. Southern, D. Tuncel, and K. T. Al-Jamal, Engineering Red-Emitting Multi-Functional Nanocapsules for Magnetic Tumour Targeting and Imaging. Biomater. Sci. 2020, 8, 2590-2599.
- S. Bozdag, K. Dillen, J. Vandervoort, and A. Ludwig, The Effect of Freeze-Drying with Different Cryoprotectants and GammaIrradiation Sterilization on the Characteristics of Ciprofloxacin HCl-Loaded Poly-(D,L-Lactide-Glycolide) Nanoparticles. J. Pharm. Pharmacol. 2010, 57, 699-707.
- J. Crecente-Campo, S. Lorenzo-Abalde, A. Mora, J. Marzoa, N. Csaba, J. Blanco, A. González-Fernández, and M. J. Alonso, Bilayer Polymeric Nanocapsules: A Formulation Approach for a Thermostable and Adjuvanted E. Coli Antigen Vaccine. J. Control. Release 2018, 286, 20-32.
- N. M. Bexiga, A. C. Bloise, A. M. Alencar, and M. A. Stephano, Freeze-Drying of Ovalbumin-Loaded Carboxymethyl Chitosan Nanocapsules: Impact of Freezing and Annealing Procedures on Physicochemical Properties of the Formulation during Dried Storage. Dry. Technol. 2018, 36, 400-417.
- S. Meulewaeter, G. Nuytten, M. H.Y. Cheng, S. C. De Smedt, P. R. Cullis, T. De Beer , I. Lentacker and R. Verbeke, Continuous freeze-drying of messenger RNA lipid nanoparticles enables storage at higher temperatures, J. Contr. Rel., 2023, 357, 149-160.
- L. Ai, Y. Li, W. Yao, H. Zhang, Z. Hu et al., Lyophilized mRNA lipid nanoparticle vaccines with long term stability and high antigenicity against SARS-CoV-2, Cell Discovery, 2023, 9:9.
- H, Muramatsu, K. Lam, C. Bajusz, D. Laczko, K. Kariko, P. Schreiner, A. Martin, P. Lutwyche, J. Heyes, and N. Pardi, Lyophilization provides long term stability of a lipid nanoparticle-formulated nucleoside modified mRNA vaccine, Mol. Ther., 2022, 30, 1941-1951.
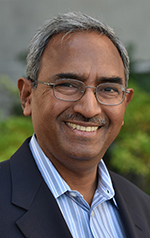
Shauket Ali, PhD
Sr. Director, Scientific Affairs & Technical Marketing
Ascendia Pharmaceuticals
shaukat.ali@ascendiapharma.com
www.ascendiapharma.com
Dr. Shaukat Ali joins Ascendia Pharmaceuticals Inc. as Senior Director of Scientific Affairs and Technical Marketing after having worked in the pharma industry for many years. His areas of expertise include lipid chemistry, liposomes, lipid nanoparticles, surfactant-based drug delivery systems, SEDDS/SMEDDS, oral and parenteral, topical and transdermal drug delivery, immediate- and controlled-release formulations. He earned his PhD in Organic Chemistry from the City University of New York and carried out his post-doctoral research in Physical Biochemistry at the University of Minnesota and Cornell University. He has published extensively in scientific journals and is inventor/co-inventor of several US and European patents.
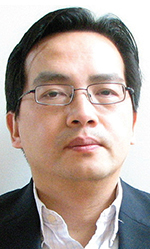
Jim Huang, PhD
Founder & CEO
Ascendia Pharmaceuticals
j.huang@ascendiapharma.com
www.ascendiapharma.com
Dr. Jim Huang is the Founder and CEO of Ascendia Pharmaceuticals, Inc. he earned his PhD in Pharmaceutics from the University of the Sciences in Philadelphia (formerly Philadelphia College of Pharmacy and Sciences) under Joseph B. Schwartz. He has more than 20 years of pharmaceutical experience in preclinical and clinical formulation development, manufacturing, and commercialization of oral and parenteral dosage forms. His research interests are centered on solubility/bioavailability improvement and controlled delivery of poorly water-soluble drugs through nano-based technologies.
Total Page Views: 2040