Issue:May 2023
FORMULATION FORUM - LIPIDSOL®: Liposomes - Chemistry, Properties & Applications of Lipid Nanoparticles
INTRODUCTION
LipidSol®, Ascendia’s lipid nanoparticle (LNP) platform technology, is aimed to improve the delivery of therapeutic modalities entrapped in lipid structures varying with different compositions of long chain fatty acids and polar headgroups. Designed specifically to increase maximum entrapment in the LNP interior, LipidSol, can adopt different shapes and sizes and can be recognized by different assemblies as shown in Figure 1.
The landscape of LNPs is continuously evolving as more and more challenging therapeutic modalities are discovered in the preclinical phase that require parenteral route of administration with controlled drug delivery.1 In a recent survey by the chemical abstract services (CAS) between 2000 and 2020, focusing on newer trends after the era of traditional liposomes, more than 1700 documents related to LNPs were published as patents and non-patented articles in journals and books (Figure 2).2 In LNP subcategories, immunogenic and cationic liposomes are the fastest growing areas followed by stealth liposomes, solid lipid nanoparticles (SLNs), nanostructured lipid carriers (NLCs), and cubosomes.
As indicated, these LNPs play an important role in the formulation and delivery of a number of modalities. Liposomes were discovered in 1965; since then, they have been used as carriers in the transport of hydrophobic and/or hydrophilic, small and large therapeutic modalities, including proteins, peptides, and nucleic acids. A number of drugs using liposome technologies have been commercialized successfully for treatment of cancers, inflammation, and other rare diseases.3 Other structural modifications of LNPs include SLNs, NLCs, immunogenic liposomes engineered with conjugated antibodies (immunogenic) for targeting diseases tissues, and lipoplexes engineered with cationic lipids for encapsulation of vaccines and biologics. Like other LNPs, the cubosomes designed with low melting lipids and polymers are also recognized for their longer circulation and effective delivery of drug molecules.4
CHEMICAL STRUCTURE OF LIPIDS
Before getting into the details of liposomes, let’s first examine the structure of these lipids, preferably the di-acyl phosphatidylcholines, wherein, the di-acyl chains at the glycerol backbone represent the lipophilicity of the lipids and the phosphatidylcholine moiety represents the polar headgroup.5 The glycerol backbone is tethered with fatty acid chains at the sn-1 and sn-2 positions, and the headgroup composed of phosphate and choline moieties are attached at the sn-3 position of the natural lipids. These groups are susceptible to membrane phospholipases; for example, the fatty acid chains at sn-1 and sn-2 are susceptible to phospholipase A1 and A2, respectively; whereas the phosphate and choline moieties are prone to hydrolysis by phospholipase C and D, respectively.6
In spite of the susceptibility to enzymatic degradations, these phospholipids are important carriers for delivery of payload through systemic circulation.7 The structural modifications of these lipids make them less susceptible for enzymatic degradation. For example, the acyl chains modified with an ether linkage can lead to longer circulation and act as an oncology drug, and may lead to greater therapeutic efficacy in encapsulated liposomes.8 An ether lipid, for example, with a O-C18 fatty acid at the sn-1 position, and an O-methyl group at the sn-2 position, is highly stable, which makes it a good candidate as “edelfosine” for treatment of certain cancers.9
LIPOSOMES AS A CARRIER FOR DRUG DELIVERY
Since its discovery in mid 1960s, liposomes have been used extensively in the formulation of many drug molecules.10 A handful of liposome-based drugs have been marketed commercially (Table 1).2 Because of their unique structures with inner aqueous cavity and a lipophilic bilayer interior, the drug molecules, amphiphilic and/or hydrophobic in nature, get preferentially partitioned into these assemblies. Thus, these lipid assemblies can act as carriers in transporting the molecules to target tissues.11 These lipid molecules used as LNPs can be designed with a wide spectrum of structures typically derived from long saturated or unsaturated fatty acid chains as the lipophilic interior and polar headgroup as the hydrophilic moieties facing the exterior aqueous phase. There have been continued interest in designing smarter molecules with a headgroup to provide and electrostatic charge to prevent flocculation or aggregation and to enable longer circulation in the blood.12 These modifications include replacing the PC headgroup with phosphatidyl ethanolamine (PE), phosphatidyl glycerol (PG), phosphatidyl serine (PS), and/or phosphatidyl inositol (PI).13 Applications of liposomes composed of phospholipids, however, could be limited by its shelf-life and its ability to protect encapsulated drugs due to high permeability leading to leakage of its payload through bilayer membranes. Thus, cholesterol or sterol is used to overcome membrane flexibility, permeability, and stability.14 Liposomes with other ingredients, such as vitamin E or Vitamin E-TPGS, PEGs incorporated in bilayers, serve as carriers in transporting and protecting the molecules from environmental pHs, enzymatic, and chemical changes as well as shielding them from temperature and ion fluctuations, thus improving the stability, shelf-life and bio-distribution.15
Phospholipids with varied chain lengths and fatty acid compositions and headgroups, like PC, PE, PG, and PS, among others, are spontaneously aggregate in aqueous solution to form lipid particles ranging between 20 nm and 1000 nm. The insoluble molecules can partition into the lipid interior if hydrophobic, or if hydrophilic, can partition in the aqueous interior of liposomes. These liposomes can be prepared via simple agitation to sonication to homogenization methods as small vesicles (SUVs) with particle size of 20-100 nm, large unilamellar vesicles (LUVs) with particle size diameter of 100-1000 nm, or multilamellar vesicles (MLVs) with diameters of >500 n. Liposome particle size is a critical attribute for drug encapsulation and circulation half-life. Smaller size liposomes circulate longer than larger sizes without being engulfed by phagocytes.16 Several drugs approved in liposomes typically have the particle size range of 100 nm or less.17 The particle size of liposomes is controlled by extrusion, sonication, and homogenization, and is measured by dynamic light scattering, size exclusion chromatography, nuclear magnetic resonance spectroscopy, and light microscopy.
Lipid headgroup plays an important role in drug delivery at the cellular level.18 The surface charges created by positive, negative, and/or zwitterionic moieties contribute to potential to cross the membrane bilayers, allow partitioning and migration of drugs from the outer to inner membranes, and the stability of liposomes, in general. For instance, liposomes with higher charges around the membrane and contributed by, for instance, PG or PE, may provide much better stability due to charge repulsions than those with neutral lipid membranes. A zeta potential -30 mV or >30 mV can lead to stronger charge repulsion and particle segregation in these suspensions than those with lower zeta potential.19
Many anti-cancer drugs are typically formulated in liposomes or LNPs due in part to most of those drugs are poorly soluble and cytotoxic. Therefore, better and efficient encapsulation methods are required to solubilize, protect, and transport these molecules to the target tissues to enhance efficacy and reduce drug toxicity. As evident from Table 1, the earliest approved drug doxorubicin was formulated in liposomes and approved for treatment of ovarian cancer followed by the approval of Epaxel in liposomes to deliver the hepatitis A vaccine earlier in 1993.20,21
CASE STUDY
In a case study, liposomes were encapsulated with carvedilol (@7%) and prepared by dissolving the drug and lipids in ethanol. In formulation F1, liposomes were composed of DMPC:Cholesterol:DSPE (52:21:22), whereas, in formulation F2, the liposomes were composed of egg PC:Chol (65:27).23 The ethanolic solution of lipid and drug was heated at 60°C, which was then injected into 0.9% saline. Following high pressure homogenization at 12,000 PSI through 10 cycles, the lipid suspensions were sterilized by filtration through a 0.22-micron PTFE filter. The free drug was removed through dialysis in 0.9% saline, and the lipid suspensions with 75-150 nm particle size were obtained by extrusion with higher encapsulation efficiency (ca. 80%-90%) and unimodal distribution (PDI 0.12-0.19).
For in vivo studies, carvedilol was single dosed at 2.5 mg/kg, and pharmacokinetic (PK) data from three animal groups (DMPC liposome formulation #1 in Group 1, Egg PC liposome formulation # 2 in Group 2, and aqueous solution in Group 3) were collected and are shown in Figure 3. In vivo data in rats following iv administration shows that drug continues to circulate longer and is maintained in plasma over 24 hours as compared to drug in aqueous solution, which is cleared out in < 4 hours.
SUMMARY
This article described the latest trends in LNPs as more and more novel therapeutic modalities discovered can’t be handled by traditional microemulsions or nanoemulsions. Ascendia’s LipidSol technology encompasses all the lipid nanoparticles to address a variety of therapeutic modalities that can’t be solubilized and delivered to diseased tissues by traditional oral, injectable, or topical routes of administration. In cases like these, LipidSol enables the encapsulation of drugs in LNPs by the utilization of fatty acids and polar headgroup entities that are commercially available and listed in the FDA inactive ingredient database (IID). In addition to traditional liposomes, other LNPs, such as immunogenic and stealth liposomes, SLNs, NLCs, and cubosomes, will play an important role as more challenging modalities are discovered. Ascendia’s footprint in innovative platform technologies, for instance LipidSol, will continue to address the challenges in injectable formulations requiring controlled delivery of small molecules, biologics, and genes.
REFERENCES
- J. Huang and S. Ali, Long-acting injectable nanoparticle formulations, Am. Pharm. Rev., 2023, March 1.
- R. Technov, R. Bird, A. E. Curtze and Q. Zhou, Lipid nanoparticles- From liposomes to mRNA vaccine delivery, a landscape of research diversity and advancement, ACS Nano, 2021, 15, 16982-17015.
- M. A. S. Boushehri, D. Dietrich and A. Lamprech, Nanotechnology as a platform for the development of injectable parenteral formulations: A comprehensive review of the know-hows and state of the art, Pharmaceutics 2020, 12, 510; doi:10.3390/pharmaceutics12060510.
- H. M. G. Barriga, M. N. Holme, and M. M. Stevens, Cubosomes: The next generation of smart lipid nanoparticles? Angew. Chem., Int. Ed. 2019, 58, 2958−2978.
- S. Ali and R. Bittman, Facile diacylation of glycidyl tosylate: Chiral synthesis of symmetric chain glycerophospholipids, J. Org. Chem., 1988, 53, 5547-5549.
- S. Cerminati, L. Paoletti, A. Aguirre, S. Peirú, H. G. Menzella and M. E. Castelli, Industrial uses of phospholipases: current state and future applications, Appl. Micro. Biotech., 2019, 103, 2571–2582.
- L. Sercombe, T. Veerati, F. Moheimani, S. Y. Wu, A. K. Sood and S. Hua, Advances and challenges of liposome assisted drug delivery, Frontier Pharmacol., 2015, 6, 286. doi.org/10.3389/fphar.2015.00286.
- E. Mayhew, A. S. Janoff, I. Ahamd, and S. Bhatia, Etherlipid containing multiple lipid liposomes, US Patent 6,180,137 (June 30, 2001).
- W. E. Berdel, Ether lipids and derivatives as investigational anticancer drugs, Onkologie, 1990, 13, 245. https://doi.org/10.1159/000216771.
- A. D. Bangham, Liposomes: the Babraham connection, Chemistry and Physics of Lipids, 1993, 64, 275-285.
- Laouini, A.; Jaafar-Maalej, C.; Limayem-Blouza, I.; Sfar, S.; Charcosset, C.; Fessi, H. Preparation, Characterization and Applications of Liposomes: State of the Art. Journal of Colloid Science and Biotechnology 2012, 1, 147−168.
- T. M. Allen, and J. M. Everest, Effect of Liposome Size and Drug Release Properties on Pharmacokinetics of Encapsulated Drug in Rats. J. Pharmacol. Exp. Ther. 1983, 226, 539−544.
- S. Vemuri and C.T. Rhodes, Preparation and characterization of liposomes as therapeutic delivery systems: a review, Pharm. Acta Helv. 1995, 70, 95–111.https://doi.org/10.1016/0031-6865(95)00010-7.
- G. Gregoriadis, C. Davis, Stability of liposomes in vivo and invitro is promoted by their cholesterol content and the presence of blood cells, Biochem. Biophys. Res. Commun. 1979, 89, 1287–1293, https://doi.org/10.1016/0006-291X(79)92148-X.
- S. Saraf, A. Jain, A. Tiwari, A. Verma, P.K. Panda, S.K. Jain, Advances in liposomal drug delivery to cancer: an overview, J. Drug Deliv. Sci. Technol. 2020, 56, 10154., https://doi.org/10.1016/J.JDDST.2020.101549.
- H. Harashima, K. Sakata, K. Funato, and H. Kiwada, Enhanced Hepatic Uptake of Liposomes through Complement Activation Depending on the Size of Liposomes. Pharm. Res. 1994, 11, 402−406.
- Nagayasu, A.; Uchiyama, K.; Kiwada, H. The Size of Liposomes: A Factor Which Affects Their Targeting Efficiency to Tumors and Therapeutic Activity of Liposomal Antitumor Drugs. Adv. Drug Delivery Rev. 1999, 40, 75−87.
- Jacques P. F. Doux, B. A. Hall, and J. A. Killian, How Lipid Headgroups Sense the Membrane Environment: An Application of 14N NMR, Biophys. J., 2012, 103, 1245–1253.
- M. C. Smith, R. M. Crist, J. D. Clogston, and S. E. McNeil, Zeta Potential: A case study of cationic, anionic, and neutral liposomes. Anal. Bioanal. Chem. 2017, 409, 5779−5787.
- P. K. Working and A. D. Dayan, Pharmacological-Toxicological Expert Report. Caelyx. (Stealth Liposomal Doxorubicin Hcl). Hum. Exp. Toxicol. 1996, 15, 751−785.
- U. Bulbake, S. Doppalapudi, N. Kommineni, and W. Khan, Liposomal Formulations in Clinical Use: An Updated Review. Pharmaceutics 2017, 9, 1−33.
- J. Nel, K. Elkhoury, E. Velot, A. Bianchi, S. Acherar, G. Francius, A. Tamayol, S. Gandemange, and E. Arab-Tehrany, Functional liposomes for targeted breast cancer drug delivery, Bioactive Materials, 2023, 24, 401-437.
- V. Ivanova, K. Maeda, W. Wang, D. Guo and J. Huang, Parenteral sustained release delivery of carvedilol disperse system, US Patent 10,792,244 (October 6, 2020).
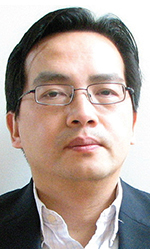
Jim Huang, PhD
Founder & CEO
Ascendia Pharmaceuticals
j.huang@ascendiapharma.com
www.ascendiapharma.com
Dr. Jim Huang is the Founder and CEO of Ascendia Pharmaceuticals, Inc. he earned his PhD in Pharmaceutics from the University of the Sciences in Philadelphia (formerly Philadelphia College of Pharmacy and Sciences) under Joseph B. Schwartz. He has more than 20 years of pharmaceutical experience in preclinical and clinical formulation development, manufacturing, and commercialization of oral and parenteral dosage forms. His research interests are centered on solubility/bioavailability improvement and controlled delivery of poorly water-soluble drugs through nano-based technologies.
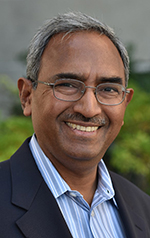
Shauket Ali, PhD
Sr. Director, Scientific Affairs & Technical Marketing
Ascendia Pharmaceuticals
shaukat.ali@ascendiapharma.com
www.ascendiapharma.com
Dr. Shaukat Ali joins Ascendia Pharmaceuticals Inc. as Senior Director of Scientific Affairs and Technical Marketing after having worked in the pharma industry for many years. His areas of expertise include lipid chemistry, liposomes, lipid nanoparticles, surfactant-based drug delivery systems, SEDDS/SMEDDS, oral and parenteral, topical and transdermal drug delivery, immediate- and controlled-release formulations. He earned his PhD in Organic Chemistry from the City University of New York and carried out his post-doctoral research in Physical Biochemistry at the University of Minnesota and Cornell University. He has published extensively in scientific journals and is inventor/co-inventor of several US and European patents.
Total Page Views: 6071