Issue:September 2024
FORMULATION DEVELOPMENT - Solubility-Enhancing Technologies in Pharmaceutical Development, a Mini-Review
INTRODUCTION
The Biopharmaceutics Classification System (BCS) and Developability Classification System (DCS) have been used since the 1990s to classify drugs into different categories based on their permeability or solubility. A frequent challenge in contemporary drug development lies in the low aqueous solubility exhibited by a significant proportion of emerging Active Pharmaceutical Ingredients (APIs), thereby negatively affecting their absorption following oral administration.
Most of the drugs in development fall into categories in which solubility poses challenges, particularly when aiming for oral administration. In the initial stages of drug development, liquid formulations are typically preferred due to their ease of modification and adaptability. However, as the development process progresses into later phases, the formulation strategy needs to shift toward more user-friendly formats, such as tablets or capsules. This transition is driven by factors such as ease of administration, patient compliance, and stability of the drug product.
To tackle these challenges, different solubility-enhancing technologies can be used by developers. With different advantages and drawbacks, linked to payload, manufacturability, tolerability, and physical and chemical stability, effectively navigating solubility-enhancing solutions is multifaceted.
UNDERSTANDING THE BIOPHARMACEUTICS & DEVELOPABILITY CLASSIFICATION SYSTEMS
The BCS was introduced in 1995 and classifies APIs into four distinct classes based on their solubility and permeability characteristics: Class I (high solubility, high permeability), Class II (low solubility, high permeability), Class III (high solubility, low permeability), and Class IV (low solubility, low permeability) providing a guideline for developers to predict the oral bioavailability of a drug.1,2
In reality, most of the absorption of oral drugs takes place in the small intestine, where drugs must first solubilize into gastro-intestinal (GI) fluids, to then cross GI membranes, to reach the systemic circulation and eventually generate the intended therapeutic effect. The DCS was introduced in 2010 as a revised version of the BCS. These modified guidelines recognize that certain drug candidates classified in BCS Class II exhibit unique challenges beyond solubility, influencing their delivery, focusing in particular on their behaviour in the GI tract. It takes the following into account:
- The use of more biorelevant media with concomitant more realistic volumes.
- The implementation of a solubility-limited absorbable dose (SLAD) because, at least for BCS Class II, solubility and permeability are compensatory.
- The expression of the dissolution rate as a target drug particle size rather than as a dose/solubility ratio.
The majority of recent drug candidates exhibiting low aqueous solubility fall into BCS Class II, posing a substantial difficulty in achieving optimal absorption after administration. A differentiation between those belonging to DCS Class IIa (Dissolution-rate limited) and those in Class IIb (Solubility-limited) can be made (Figure 1). Because of this, the integration of solubility-enhancing technologies has become central in pharmaceutical development strategies. The selection of a formulation approach necessitates thoughtful evaluation of various parameters, including API solubility, not only in aqueous media and representative dissolution mediums, but also in alternative solvents for administration or for the manufacturing steps. This assessment should also encompass considerations related to the physicochemical profile, permeability, and intended dose, as well as factors pertaining to manufacturing.3
EXPLORING SOLUBILITY-ENHANCING TECHNOLOGIES FOR DRUG SOLUBILITY
A wide variety of technologies (Table 1) exist to tackle poor solubility, and in general, these focus on optimizing the API itself (eg, pro-drug formation, use of different salts, co-crystallization) or by means of various formulation approaches through for instance optimization of API solubility in aqueous medium (pH-shift, surfactant-addition), suspensions associated with particle size reduction (nanosuspensions), predissolved forms (lipid-based and related systems, cyclodextrin complexes, co-solvents) or use high-energy solid forms to generate supersaturation (amorphous solid dispersions).
In preclinical toxicity testing, achieving systemic exposures at doses significantly exceeding the anticipated clinical dose is necessary, emphasizing the need for higher solubility. To reach these elevated doses, oral gavage administration is the most direct and in rodent studies, often the only option. In the early phases of clinical studies, particularly in Phase 1, clinicians prefer dosage forms that offer flexibility to cover a broad dosing range. Considering these factors of dose and administration flexibility, solubility-enhancing formulations in early development are typically liquid forms. In this respect, solutions or suspensions allow easy dilution to lower concentrations or variable volumes for achieving diverse doses.6
Practically all widely used solubility-enhancing technologies, such as nanosuspensions, lipid-based systems, and cyclodextrin complexes, can be readily processed into liquid formulations. Amorphous solid dispersions, despite being solid products, can be formulated into a suspension by dispersing them in an aqueous or alternative vehicle shortly before administration (with precautions in place to ensure this dispersion process doesn’t adversely impact its performance as dissolution of the amorphous API has already started).3
UTILIZING NANOSUSPENSIONS TO IMPROVE DRUG SOLUBILITY
Nanosuspensions represent an attractive way to improve bioavailability of poorly soluble drugs, by enhancing the dissolution rate of APIs through an increased surface area, enabling paracellular transport or lymphatic transport. Nanosuspensions can generally facilitate high payloads and require low excipient concentrations. However, they also exhibit challenges related to physical stability, such as crystal growth (also known as Ostwald ripening) and agglomeration, especially during manufacturing or long-term storage.
From a manufacturing point of view, both bottom-up and top-down approaches are possible. The bottom-up approach involves the controlled precipitation of the API from a (non-aqueous) solution or melts into a non-solvent, often in parallel with high-energy methods (eg, high-shear mixing or ultrasonication), to produce nanoparticles. This bottom-up approach enables precise control over particle size distribution during the nanoparticle formation process, contributing to a better shape and size uniformity (ie, a lower Polydispersity Index) and increased physical stability.
Alternatively, the top-down approach involves applying mechanical forces to break down larger API particles into nanosized ones using techniques like high-pressure homogenization or through wet or dry (ie, jet milling) techniques using coarse materials. Top-down is generally considered more scalable and adaptable to a larger variety of APIs, as it does not rely on solubilizing the API at any point during manufacturing.
In the formulation development stages, the objective is to ensure the long-term stability of nanosuspensions, and during this process, the identification of an effective excipient mix (eg, surfactants, polymers like polyvinylpyrrolidone) is undertaken to manage, in particular, correct size-reduction and undesirable particle growth.
The performance of nanosuspensions predominantly relies on their dissolution rate enhancement. While suitable for a diverse range of drug candidates, they may prove inadequate when formulating exceptionally challenging molecules, leading to considerations of alternative strategies, such as lipid-based systems or amorphous solid dispersions.7
HARNESSING LIPID-BASED FORMULATIONS
The use of lipid-based and related formulations, including lipid solutions, emulsions, and self-emulsifying (micro or nano) drug delivery systems (SEDDS, SNEDDS, SMEDDS), and solutions employing co-solvents and surfactants, address challenges associated with the slow dissolution of APIs from the crystalline form in aqueous media. This approach presents the API to GI fluids after administration in a pre-dissolved state in lipophilic materials, enhancing solubility and absorption, and eventually improving bioavailability. SEDDS, for instance, are a sort of (semi-)solid or liquid formulation composed of the following:
- The API,
- Oils (medium-chain triglycerides eg, caprylic acid, capric acid, lauric acid; long-chain triglycerides, eg, soybean oil, sunflower oil, mixes of medium and long-chain triglycerides; others, eg, isopropyl myristate),
- Waxes/fats (eg, gelucire),
- Surfactants (eg, sodium lauryl sulfate, sorbitan monostearate, lecithin),
- Humectants (eg, glycerol, sorbitol, polyethylene glycol/PEG), and
- Co-solvents on an occasional basis (eg, ethanol).
These SEDDS form in situ emulsions with moderate stirring and dilution by water-phase of GI fluids directly after administration in the GI tract. As such, they can increase the solubility and bioavailability of poorly soluble APIs.
Challenges associated with this approach include potential limitations on achievable payloads, particularly for APIs with high melting points or with equally low solubility profiles in lipids. For SEDDS for instance, high payloads can only be achieved if the API demonstrates a remarkable solubility in one of the excipients used (eg, oil or surfactant). Moreover, lipid formulations often incorporate excipients containing reactive molecules or impurities (eg, esters, formaldehyde, peroxides), posing risks of chemical degradation such as oxidation, acylation, and transacylation, which can be critical for the stability and safety of the formulation.
These stability issues can be mitigated by the formulators through careful choice of excipients, or by presenting the API in a dried form, to be reconstituted with the lipidic vehicle right before administration (eg, two vials presentation). Risks of precipitation upon dilution in vivo or of poor release from the emulsified systems also exist and should not be overlooked as they could impact bioavailability. Finally, a major drawback of these formulations is their poor palatability and the taste of some excipients (eg, surfactants). After early phase studies where this is often inevitable, taste-maskers (eg, sweeteners, flavorants) or liquid-filled capsules (eg, soft gels or hard caps) are often chosen.8,9
MAKING CYCLODEXTRIN COMPLEXES TO PROMOTE SOLUBILITY
Cyclodextrins have been widely used as pharmaceutical excipients since the 1980s due to manufacturing improvements. They are oligosaccharides composed of between 6-8 α-(1,4) linked α-D-glucopyranose units arranged in a ring. Their inner cavity is therefore lipophilic, while their outer surface is hydrophilic, which makes them soluble in water, and able to “shield” lipophilic API from the aqueous media, enhancing the API solubility.
Hydrophilic derivatives of β-cyclodextrins, such as hydroxypropyl-β-cyclodextrin (HP-β-CD), are very well characterized and have low toxicity (ie, up to approx. 28 g β-CD can be dosed per day). Cyclodextrins offer enhanced stability against dilution-induced precipitation, setting them apart from alternative solubilization techniques like cosolvents. Their ability to efficiently and specifically bind with the API enhances solubility in aqueous media in a targeted and effective manner, providing a tailored solution for specific drug compounds with high complexation profiles.
However, cyclodextrin-based formulations typically face payload restrictions, rarely exceeding 5%. One significant disadvantage arises in the context of toxicity studies, particularly in rodents. The payload restrictions associated with cyclodextrins may limit their extensive use in such studies in which higher drug concentrations are often necessary for accurate assessments. This limitation can impact their applicability. Additionally, the cost-effectiveness of cyclodextrin-based solubilization should be carefully evaluated, considering their very high cost.
Considering scalability is crucial for the broader adoption of cyclodextrin-based solubilization processes. Furthermore, understanding how frequently this approach is applied in contemporary pharmaceutical development strategies provides insights into its practicality on a larger scale.10-12
PRODUCING AMORPHOUS SOLID DISPERSIONS
Amorphous solid dispersions (ASDs) are based on the molecular dispersion of the API, which corresponds to the disruption of the crystalline lattice, into an inert carrier such that less energy is required to promote their dissolution. As a result, ASDs present an interesting solution in drug development of poorly soluble APIs offering a significant advantage in circumventing bioavailability issues. The amorphous state of the dispersion enhances the rate and the extent of dissolution of the API, as it enables and extends supersaturation (a so-called spring- and parachute effect) in the GI tract, enhancing bioavailability.
However, ASDs manufacturability is often complex. The production process requires the use of either organic solvents (eg, in spray drying) or high temperatures (eg, in hot-melt extrusion), introducing challenges related to API degradation, process control, reproducibility, and consistency. This can potentially impact the ability to go to large-scale manufacturing.
A key challenge in the development of ASDs is stability, both chemically and physically, upon storage. The inherent instability of the amorphous state makes it susceptible to transitioning into a crystalline form over time. Preventing and reliably detecting crystallization therefore becomes a critical aspect of the development process. Prevention starts from a thorough ASD formulation and excipient selection process (eg, polymers, surfactants), as they can help to stabilize the amorphous state by interacting with the API. Other parameters, such as controlling the storage conditions (ie, temperature and humidity), are also key in that aspect. In addition, having the correct tools at your disposal to assess the solid state of these systems enables early detection of the onset of crystallization and hence high-quality selection of concepts.13
SUMMARY
In summary, addressing the challenge of low aqueous solubility in drug development requires a comprehensive evaluation of API properties and solubility-enhancing technologies (Figure 2). Beyond increasing solubility, factors like payload, manufacturability, administration convenience, and stability play pivotal roles in shaping strategic decisions during early drug development.
As drug development advances into later phases, the selected formulation strategy also needs to evolve from liquid formulations, valuable in the early stages, to tablets or capsules.
Among the array of solubility-enabling technologies, nanosuspensions, lipid-based formulations, cyclodextrins, and ASDs each present distinct advantages and challenges. The choice of technology will depend on the unique characteristics of the drug candidate and the requirements of the development phase.
REFERENCES
- Amidon G, Lennernäs H, Shah V, Crison J. A theoretical basis for a biopharmaceutic drug classification: the correlation of in vitro drug product dissolution and in vivo bioavailability. Pharm res. 1995 Mar;12(3):413-20. doi: 10.1023/a:1016212804288.
- FDA, M9 Biopharmaceutics Classification System – Guidance for Industry, 2021.
- Butler J, Dressman J. The Developability Classification System: Application of Biopharmaceutics Concepts to J Pharm Sci. 2010 Dec;99(12):4940-54. doi: 10.1002/jps.22217.
- Nikolakakis I, Partheniadis I. Self-Emulsifying Granules and Pellets: Composition and Formation Mechanisms for Instant or Controlled Release. Pharmaceutics. 2017 Nov 3;9(4):50. doi: 10.3390/pharmaceutics9040050.
- Samineni R, Chimakurthy J, Konidala, S. Emerging Role of Biopharmaceutical Classification and Biopharmaceutical Drug Disposition System in Dosage form Development: A Systematic Review. Turk J Pharm Sci. 2022 Dec; 19(6): 706–713.
- Buckley S, Frank K., Fricker G, Brandl M. Biopharmaceutical classification of poorly soluble drugs with respect to “enabling formulations”. Eur J Pharm Sci. 2013 Sep 27;50(1):8-16. doi: 10.1016/j.ejps.2013.04.002.
- Patel V, Agrawal Y. Nanosuspensions: An approach to enhance solubility of drugs. J Adv Pharm Technol Res. 2011 Apr-Jun; 2(2): 81–87. doi: 10.4103/2231-4040.82950.
- Zhu Y, Ye J, Zhang Q. Self-emulsifying Drug Delivery System Improve Oral Bioavailability: Role of Excipients and Physico-chemical characterization. Pharm Nanotechnol. 2020;8(4):290-301. doi: 10.2174/2211738508666200811104240.
- Salawi A. Self-emulsifying drug delivery systems: a novel approach to deliver drugs. Drug Deliv. 2022 Dec;29(1):1811-1823. doi: 10.1080/10717544.2022.2083724.
- Jambhekar S, Breen P. Cyclodextrins in pharmaceutical formulation I: Structure and physicochemical properties, formation of complexes, and types of complex. Drug Discov Today . 2016 Feb;21(2):356-62. doi: 10.1016/j.drudis.2015.11.017.
- Kiss T, Fenyvesi F, Bácskay I, Váradi J, Fenyvesi E, Iványi R, Szente L, Tósaki A, Vecsernyés M. Evaluation of the cytotoxicity of B-cyclodextrin derivatives: Evidence for the role of cholesterol extraction. Eur J Pharm Sci . 2010 Jul 11;40(4):376-80. doi: 10.1016/j.ejps.2010.04.014.
- Fenyvesi F, Phuong Nguyen T, Haimhoffer A, Rusznyák A, Vasvári G, Bácskay I, Vecsernyés M, Ignat SR, Dinescu S, Costache M, Ciceu A, Hermenean A, Váradi J. Cyclodextrin Complexation Improves the Solubility and Caco-2 Permeability of Chrysin. Materials (Basel) . 2020 Aug 16;13(16):3618. doi: 10.3390/ma13163618.
- Bhujbal S, Mitra B, Jain U, Gong Y, Agrawal A, Karki S, Taylor L, Kumar S, Zhou S. Pharmaceutical amorphous solid dispersion: A review of manufacturing strategies. Acta Pharm Sin B . 2021 Aug;11(8):2505-2536. doi: 10.1016/j.apsb.2021.05.014.
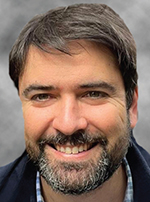
Dr. Vincent Levet is Director, Formulation Development and Production, Ardena. He earned his MPharm and PhD in Pharmaceutical Technology from ULB, Belgium, with an MBA from Quantic, USA. His expertise spans from developing sustained-release solid lipid microparticles for lung cancer treatment to leading formulation teams at GSK Vaccines and Lonza Drug Product Services. Since September 2023, he has directed Formulation Development and GMP Production at Ardena, guiding a diverse team to excellence in early-phase clinical trials.
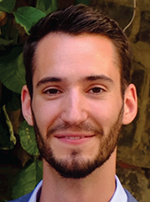
Dr. Timothy Pas is Group Leader, Formulation Development and Production, Ardena. He earned his Master’s in Pharmacy and his PhD in Pharmaceutical Technology from KU Leuven, specializing in enabling technologies and galenic principles. Transitioning to industry at Ardena, he progressed from Scientist to Group Leader, excelling in solid and liquid oral formulations, enabling technologies, and galenic presentations. Leading a talented team, he drives drug development and GMP manufacturing of early stage clinical materials, showcasing Ardena’s commitment to pharmaceutical innovation.
Total Page Views: 3640