Issue:July/August 2013
FORMULATION DEVELOPMENT - Amorphous Dispersion Formulation Development: Phase-Appropriate Integrated Approaches to Optimizing Performance, Manufacturability, Stability & Dosage Form
ABSTRACT
Given the ever-increasing number of compounds that present oral absorption challenges, efficient and “phaseappropriate” formulation development is crucial for robust optimization of key formulation attributes and successful progression of these compounds. Amorphous dispersions enable progression of low-solubility compounds and open multiple opportunities for optimization and understanding during formulation and process development. Deep understanding of the critical-to-performance attributes of amorphous dispersions as they relate to performance, manufacturability, stability, and downstream manufacture into solid dosage forms makes efficient and robust formulation development possible.
This paper discusses the development and use of amorphous spray-dried dispersions (SDDs) and the testing methodologies used to optimize the formulation and spray-drying process for performance, manufacturability, stability, and incorporation into final dosage forms. Innovative models and tools are used to predict and select the optimum formulation and process early in the development process. Use of guidance maps, process development flowcharts, in vitro tests, and models all enable efficient and successful formulation development.
INTRODUCTION
Amorphous SDDs provide multiple opportunities for co-optimization of performance, manufacturability, and stability during formulation development. Process and formulation development are inherently tied to the end goal of developing a robust formulation that can meet the desired bioperformance targets, maintain adequate physical stability, and enable a path to future scale-up (Figure 1). Use of “phase-appropriate” formulation and development models, both experimental and in silico, can be coupled to identify the overlap of process and formulation space.
A previous article contributed by Bend Research was focused on amorphous SDDs, a widely applicable platform technology that has precedence from discovery through commercialization, to improve solubility.1 Use of guidance maps and determination of critical-to-performance attributes can enable rapid selection of lead SDD formulations for progression of compounds. Rational selection of in vitro techniques to make informed decisions about selection of formulations and the process space can be conducted in a phase-appropriate manner.
PERFORMANCE
Use of SDDs to address the solubilization problems associated with DCS Class IIa/b compounds requires development of a deep understanding of the critical-toperformance attributes for specific drug molecules.2 Evaluation of key molecular properties and the use of guidance maps based on the physicochemical properties of the molecule streamline formulation screening to enable lead formulation selection. In addition, use of innovative and mechanistic in vitro tests to generate inputs for in silico models provides efficient feedback for definition of critical-to-performance attributes.
Definition of the key problem statement for each unique compound, based on the properties of the compound and target dose, is important to achieve the desired performance attributes. Typically, the primary goal with SDDs is to increase the bioavailability of insoluble compounds by raising the concentration of free drug. Additional delivery challenges and problem statements that are commonly encountered include the following:
1. Dissolution Rate – Dissolution rates are limited for compounds with high lipophilicity. Polymer selection and the addition of surfactants can be critical, as can optimization of SDD particle size.
2. Sustainment – Crystallization of soluble drug, which leads to decreased free drug and active species, must be avoided. The active loading in the SDD formulation may be limited.
3. Activity of Nanospecies – Free-drug levels and ability of colloidal and micelle structures to source free 32 drug must be evaluated. Nanoparticle formation may be critical to the performance of the SDD.
4. Precipitation During Gastric-to- Intestinal Transfer – Precipitation may occur due to high supersaturation for drugs with high solubility in gastric media during transfer to intestinal media. SDDs are formulated to minimize solubility in low-pH gastric media with enteric polymers to sustain the active species of drug in higher-pH intestinal media.
Based on the absorption challenges for the specific molecule, in vitro assays that indicate bioperformance are used orthogonally to understand the key species present and to ensure a robust formulation. Table 1 lists the specific in vitro tests and key formulation properties used to assess the various processes and physicochemical properties that are important during final dissolution of the formulation into critical active species that will enable absorption.
Each test specifically studies the kinetics of formation of one or more of the following: (1) active species that are present in solution, including free drug; (2) drug in bile-salt micelles; and (3) activity of the remaining solid or precipitate formed during the test. Outputs from these tests are also used as inputs into predictive biomodels that guide formulation selection and optimization.
Previous publications have discussed use of the DCS system and formulation maps to guide formulation of SDDs.3 These approaches streamline formulation selection by using historical experience to evaluate a small number of formulations that are likely to solve the key challenges for a given drug compound and to select the most appropriate in vitro tests. Mechanistic understanding of the key processes is critical to predicting how the formulation will perform in vivo and solving delivery challenges.
MANUFACTURABILITY
The deep understanding and optimization of the SDD formulation is then combined with careful process development based on engineering fundamentals. This approach results in a robust, high-performance drugproduct intermediate that can be manufactured using a scalable process.
The SDD technology and spray-drying process are widely applicable from early stage discovery to formulation development to commercialization. Figure 2 provides an overview of the standard spray-drying process train and typical batch quantities, comparing the different scales of equipment used at each development phase.
Optimization of the spray-drying manufacturing process is straightforward using a fundamental engineering approach. The goal is to demonstrate a robust process space that can be easily scaled. A rational flowchart methodology based on this approach can be applied to optimize the spray-drying process, focusing on two core processes: atomization and drying.4
Impacts of the process on critical formulation attributes must be considered during development to ensure the formulation is amenable to future scale-up. The two key processes — atomization and drying — both require a fundamental understanding of the following potential impacts on the SDD formulation:
1. Atomization – More energy is required as the spray-dryer scale increases to maintain the same droplet size and, thus, particle size. It is important to understand the atomization limitations to ensure an equivalent particle size can be produced at larger scales, particularly for high-lipophilicity compounds for which dissolution rate is a critical-to-performance attribute.
2. Recycling of Drying Gas – Closedloop operation of the spray dryer, which uses a condenser to control the amount of residual solvent in the incoming drying gas, leads to slower drying rates for the SDDs. Slower drying rates impact the final residual-solvent content and compressibility of the SDD particles, which can impact physical stability and manufacturability of the final dosage form. Glasstransition temperature (Tg), a key physical-stability indicator, can be measured as a function of residualsolvent content to predict a process operating space that poses a low risk for physical instability.
3. Densification of Atomized Spray Plume – Higher mass fluxes of droplets through a similar contact area also contribute to slower drying rates. Understanding this change across spray-dryer scales makes it possible to predict operating-space constraints related to physical stability and SDD compressibility for manufacture of the final dosage form.
STABILITY
The physical stability of SDDs is maintained during the spray-drying process through the rapid drying kinetics of highsurface- area droplets. Rapid quenching of the droplets to a low-mobility state through use of high-Tg polymers is critical to achieving homogeneous amorphous dispersions. Because SDDs are formulated such that physical stability is dependent on molecular mobility rather than thermodynamic miscibility of the formulation components phase-appropriate tools can be selected during formulation optimization to ensure that a robust formulation and process are selected, as shown in Figure 3. It is important to consider the failure mechanisms that may be encountered during the manufacture and storage of SDDs to ensure that the appropriate formulation and in-process controls are used. Formulations with a propensity for crystallization or amorphous phase separation can be identified using characterization techniques, including modulated differential scanning calorimetry (mDSC), powder x-ray diffraction (PXRD), isothermal calorimetry, scanning electron micrography (SEM), and dissolution performance tests.
Initially, Tg can be measured as a function of relative humidity (RH) to estimate appropriate storage conditions when crystallization is considered a risk (Figure 4). Additionally, this simple test can be applied to the solvent-wet SDD during the spray-drying process to constrain the operating space to a robust region and to select secondary-drying conditions for removal of residual solvent.
For quantitative prediction of shelf-life stability, semiempirical approaches can be used with extrapolation to determine crystallization risk for storage hold times and conditions (Figure 5). Isothermal calorimery, ie, thermal activity monitoring (TAM), is useful for rapidly extrapolating physical stability by predicting crystallization kinetics because results have been consistent with accelerated and real-time stability data.5 This approach enables selection of a physically stable SDD formulation and appropriate inprocess hold times, as well as final packaging configurations based on the Tg of the SDD.
FINAL DOSAGE FORM
SDDs can be incorporated into a variety of dosage forms while maintaining their performance and stability attributes. SDD particle properties can be optimized for dosage-form manufacturing to provide additional flexibility in compression profiles and performance.
Phase-appropriate selection of dosage forms provides flexibility for early phase toxicology and clinical studies, while providing a starting point for development of dosage forms that are suitable for scale-up and commercialization. Sachet or suspension formulations are often employed to support toxicology through Phase I studies. These dosage forms can be rapidly formulated and prepared extemporaneously. Formulated solid dosage forms, including tablets and capsules, are primarily used for immediate-release (IR) formulations — the most common application of SDDs. Additionally, controlled-release (CR) formulations, including osmotic (eg, swellable-core technology) and hydrophilic matrix tablets, can be designed to modulate the delivery rate of molecules with SDD technology.
Downstream formulation of SDDs into IR formulations suitable for in vivo dosing are designed to deliver native SDD particles within the upper small intestine to maximize the absorption window. In tablet formulations, for which SDDs are incorporated into granules to enhance manufacturability, rapid disintegration (eg, less than 5 minutes) i desired to promote breakdown of the tablet into granules and subsequent rapid dissolution into native SDD particles and finally highactivity species or free drug (Figure 6). Formulations are optimized to maintain equivalent dissolution profiles and thus bioperformance of the tablet, granules, and native SDD to ensure performance is maintained over the wide range of biorelevant conditions that the dosage form will encounter during in vivo dosing. Additionally, rapid disintegration of the dosage form is achieved in either gastric or intestinal fluid to promote performance robustness.
As shown in Figure 7, key properties of the SDD formulation can be optimized around the downstream dosage-form manufacturing process. Compressibility and particle size of the SDD are defined to allow for adequate compressibility and performance of the final dosage form. Dry granulation is commonly employed to enhance the flow characteristics of the tablet formulation and allows for incorporation into both tablets and capsules.
SUMMARY
SDDs enable the evaluation and progression of low-solubility compounds throughout the development and lifecycle of a molecule. A “phase-appropriate” approach for formulation development relies on understanding the key attributes and problem statements for the given compound and approaches based on deep science and engineering fundamentals. Use of guidanc maps, complementary in vitro tests, and biomodels promotes efficient optimization and inherent robustness of the formulation. Identification of critical-to-performance attributes for the specific compound makes it possible to employ effective testing strategies that ensure SDD formulation performance and stability attributes will be maintained. The spray-drying process can also be designed to accommodate formulation needs and produce engineered particles amenable to a wide range of solid dosage forms and sachet approaches for in vivo delivery. By considering the interplay of these different considerations during formulation selection and process development, low-solubility compounds can successfully be advanced and positioned for subsequent scale-up activities. Early integration of performance, manufacturability, stability, and dosage-form selection can be used to identify and mitigate potential risks during scale-up, leading to efficient compound advancement.
REFERENCES
1. Bloom CJ, Lyon DK. Formulation selection: amorphous dispersions & other tools for drug discovery formulation support. Drug Dev Delivery. 2013;13(3):32-37.
2. Butler J, Dressman J. The developability classification system: application of biopharmaceutics concepts to formulation development. J Pharm Sci. 2010;99:4940- 4954.
3. Friesen DT, Shanker RM, Crew MD, Smithey DT, Curatolo WJ, Nightingale JAS. Hydroxypropyl methylcellulose acetate succinate-based spray-dried dispersions: an overview. Mol Pharm. 2008;5(6):1003-1019.
4. Dobry D, Settell DM, Baumann JM, Ray RJ, Graham LJ, Beyerinck RA. A modelbased methodology for spray-drying process development. J Pharm Innovat. 2009;4(3):133-142.
5. Bloom CJ, Marsac P, Jordan FD, Sather CA, Vodak DT, Lowinger M, Liu HXi, Lupton L. Prediction of crystallization kinetics for a spray-dried dispersion using isothermal calorimetry. Poster 3293 presented at the American Association of Pharmaceutical Scientists (AAPS) Annual Meeting and Exposition, Chicago, Illinois, October 14-18, 2012.
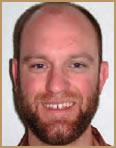
John Baumann is the Director of Spray-Dried Particle Engineering at Bend Research, where he has worked since 2004. Mr. Baumann manages a spraydrying process-development group focused on the preparation of pharmaceutical intermediates and the scale-up and transfer of spray-drying processes from research laboratories to production in a current Good Manufacturing Practice (cGMP) environment. He holds a bachelor’s degree in Bioengineering from Oregon State University.
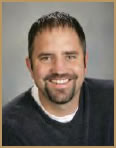
Dan Dobry, a Vice President at Bend Research, leads a group focused on formulation and process engineering for novel drug-delivery technologies. In addition to business development and client relationship management, Mr. Dobry’s responsibilities include managing the process-engineering group, which is focused on formulation and process engineering for novel drugdelivery technologies. He has worked for Bend Research since 1998 and holds a bachelor’s degree in Chemical Engineering from Oregon State University.
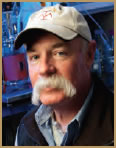
Dr. Rod Ray is the CEO and Chairman of the Board of Directors for Bend Research Inc., a world-class independent scientific development and manufacturing company. A Bend Research employee since 1983, Dr. Ray has led the company from a small contract R&D firm to a company with six facilities in Bend, Oregon, and nearly 300 employees. He holds a bachelor’s degree from Oregon State University and master’s and doctoral degrees from the University of Colorado, all in Chemical Engineering.
Total Page Views: 3282