Issue:April 2021
EMULSIFICATION TECHNOLOGY - Microspheres for Sustained Release
INTRODUCTION
Continued growth in interest in biodegradable polymers, stretching across the biomedical community for more than 50 years, has resulted in significant biotechnology advances in drug delivery, biomaterials, tissue engineering, and medical device development. Born out of interdisciplinary collaborations of individuals across the globe from fields as diverse as chemistry, engineering, biology, and physics, these researchers’ shared interests in drug development have resulted in discoveries of more potent therapeutics in the form of peptides, proteins, nucleic acids, and other bioactive molecules. These therapies, more fragile than older approaches, require careful formulation of the drug delivery vehicle if they are to remain therapeutically intact with good economics until they perform their therapeutic purpose.
Popular drug delivery vehicles include lactide and glycolide homo- and copolymers because they can be fabricated into a variety of morphologies, including nano- and microspheres, thereby enabling highly tailored and versatile controlled and long-acting release to better control therapeutic outcomes. While not the preferred route of drug delivery, their main advantages are that they can deliver therapies directly to a target site and/or ensure better patient compliance over a prolonged time period.
Administration of drugs in the form of microspheres generally enhances the outcome through isolation of the given API at, or close to, the site of action and by extending drug release. Moreover, more delicate drugs, including peptides and proteins, can be protected against chemical and enzymatic degradation when entrapped in microspheres. Poly(lactic acid) (PLA), poly(lactic-co-glycolic acid) (PLGA), and poly-ε caprolactone(PCL) are among the well-documented FDA-approved polymers used for the preparation of safe and effective vaccine, drug, and gene therapy delivery systems using well-described reproducible methods of fabrication.1 Over recent years, InnoCore Pharmaceuticals of The Netherlands has developed copolymers, such as the SynBiosys range of multi-block polymeric materials, in an attempt to enhance control over physico-chemical and degradation characteristics.
It is noteworthy that biocompatibility is not only dependent on the polymer’s intrinsic property, but also the particulate type and the biological environment. Hence, intensity and length of specific polymer-tissue interactions can be varied greatly in different organs, tissues, and species. The release of different drugs from PLA or PLGA matrices is the sum of surface and bulk diffusion as well as matrix erosion mechanisms, usually with a high initial burst rate.2
The advantages of long-acting formulations include enhanced patient compliance and convenience, and a lower dose of drug compared with a daily oral regimen. Despite these advantages, it is interesting to note that the limited number of different drug products placed on the market in the past 30+ years suggests that development of injectable, long-acting depot formulations is both demanding and challenging. What makes the development of long-acting PLGA for mulations so challenging is down to several factors.
FORMULATION REQUIREMENTS
Formulating a sustained-release dosage form demands a clear understanding of the physicochemical and biological properties of the given polymer and the polymer’s interaction with the API, with both married to an appropriate microsphere manufacturing technique. The design of a suitable morphology of porous microspheres of biopolymer to achieve the desired functionalities requires control over their physical properties, such as pore size, porosity, and microsphere size.
If required, templating methods can be used to create accurately sized porus structures. A variety of porogens, such as bovine serum albumin (BSA) and alginate microspheres, have been cited as suitable.3 One important determinant of the porosity of the microstructure is the molar ratio between lactide and glycolide in PLGA. Also, higher molecular weight PLGAs are preferred for creating a more porous structure while low molecular weight PLGAs reduce microsphere size. As well as controlling for porosity and pore size, variations of these elements is one factor in determining the encapsulation efficiency of the chosen drug within the PLGA matrix, although higher encapsulation efficiency values are achieved by production using a double emulsion technique
A well-recognized issue with PLGA microspheres is their tendency to have a high initial burst release of drug. Typically 60% of drug loading is released in the first 24 hours. Generally undesirable, this effect has been known to produce toxic levels of API in plasma, with detrimental effects. This burst release is largely due to surface porosity and can be modified, for example, by the addition of buffer to achieve a plasticising “skin” on the PLGA or through storage at high humidity to “seal” the surface.4 Another researcher has demonstrated the use of alginate coating to achieve the same effect.5 The concentration of BSA has a significant influence on the pore size. During the formation of double emulsions, the osmotic pressure drives water penetration from the external water phase into internal water phase, leading to the formation of interconnected pores and finally, porous PLGA spheres after solvent evaporation. It is, therefore, reasonable to expect smaller pores to have a lower osmotic pressure, corresponding to a lower BSA concentration in the internal water phase.6
As described earlier, the final determinant of desired functionality is control over microsphere size during a robustly scalable manufacturing method. A good manufacturing technology will also allow the attributes of the chosen polymer system to deliver optimal encapsulation efficiency values while controlling burst release.
Sterilization carried out on the finished microspheres can lead to changes in the expected performance. For this reason, an aseptic process for microsphere production is desirable.
MICROSPHERE PRODUCTION
The customary production method, the solvent evaporation process, necessitates emulsification. There are two specific forms of emulsion processes: single emulsion and double emulsion. With the single emulsion process, an appropriate amount of PLGA is dissolved in an organic solvent, eg, dichloromethane, containing the API. The solution containing API and polymer is then subsequently emulsified by addition to aqueous solution containing surfactant or emulsifying agent to form an oil-in-water emulsion.
After formation of the stable emulsion, the organic solvent evaporates, which can be accelerated by continuous stirring, dilution, adjustment of temperature or reduction of pressure. As it evaporates, the dissolved polymer precipitates to transform the droplets of polymer into solid microspheres. The microspheres thus developed are then sieved, washed, and lyophilized under sterile conditions, resulting in a final microsphere drug product suitable for fill-finish processing.
In the double emulsification process, water-soluble drugs are initially encapsulated by creating a primary emulsion of aqueous API solution in solvent+polymer solution. The resulting water-in-oil emulsion is then emulsified into aqueous buffer/surfactant solution to produce the secondary emulsion. The particle size and encapsulation efficiency of the system is controlled by solvent and stirring rate, among other factors.
Solvent extraction kinetics are vital for creating a secure shell that depends on the solvent type used, temperature, and the type of PLGA. PLGA-solvent interaction also affects the PLGA coalescence and shell formation, determining the quality of microparticles formed. Crucially, the choice of solvent determines the drug solubility in the PLGA-drug mixture, particularly for hydrophobic drugs, and therefore affects drug loading, encapsulation efficiency (EE), and drug-release kinetics. As a result of each drug’s unique physicochemical properties, each drug formulation needs a holistic consideration of PLGA specification, solvent type, and microparticle manufacturing conditions.
During the production of PLGA microspheres, slight changes in the formulation parameters can have radical effects on microsphere morphology and, in turn, drug release. With respect to double emulsion-solvent extraction, the mechanisms controlling microsphere internal porosity have been established for some time. In this instance, internal porosity is dependent on the stability of the primary emulsion.
Traditionally, manufacturing methods have relied on high shear homogenization. This gives rise to a number of inefficiencies. The significant disruption of the secondary emulsion during emulsion manufacture results in low EE values. Additionally, this technique gives a wide size distribution of microspheres, which must be sieved, with significant waste arising, to give the correct size for administration. If this technique is applied to a sensitive API, the high shear will degrade a significant fraction rendering it therapeutically useless while adding to the regulatory compliance burden.
More recently, manufacture of highly precisely sized microspheres has been developed using microfluidics technologies. Much has been written about these techniques, but they are all challenged when it comes to scale-up. The scale-up method of choice is to number up with consequently increased stringency of pumping and control systems and with no economies of scale benefits.
A third, well-established method that has the benefits of both homogenization and microfluidics but with few of their drawbacks offers precision at scale. Membrane emulsification was identified in Japan in 1985 but has come of age in the hands of Micropore Technologies Ltd.
Micropore harnesses the well-established solvent evaporation method of production with its multi-award-winning membrane emulsification technology. Micropore’s precision engineered membrane emulsification technology robustly and reliably delivers a predictably narrow size distribution (coefficient of variation <15%) at a tuneable size between 5 to 500 μm through their precision-engineered, GMP-compliant technology.
Client data highlights that it is common for 30% or more of microspheres produced using the traditional homogenization method to be over- or under-size and require removal through laborious and time-consuming sieving. The company has examples in which the material discard rate has been as high as 80% to 90%. Micropore’s technology reduces this discard to zero, with consequent improvements in processing time and economics.
While biopolymers, such as PLGA, are most commonly associated with micron-size microspheres, PLGA nanospheres are emerging as interesting drug delivery vehicles. Such is the adaptability of Micropore’s technology that it can be turned to the manufacture of this size of material.
The shear forces used in membrane emulsification are less than 50% of those required to lyse viable cells. This gentle process results in almost no degradation of sensitive APIs, retaining well above 90% biological activity compared with 37% to 67% degradation when rotor-stator homogenization is the method chosen for microsphere encapsulation.7 Because Micropore’s process is an inherently gentle process, the result is extremely high-quality microspheres when using a double emulsion process, as the primary emulsion is not broken during secondary emulsification. A gentle process enables enhanced microspheres, with high encapsulation efficiency of up to 99%, to be developed using a double emulsion-based membrane emulsification method.8
The simplicity and flexibility of Micropore’s technology is such that developments can be transferred seamlessly from preclinical formulation development on the laboratory bench, through pilot scale and into full-scale GMP manufacturing, opening opportunities for more rapid development to explore the best way to optimize bioavailability for formulations.
Of course, reproducibility is vital in a full-scale manufacturing environment. In this respect the precision-engineered solution offered by Micropore excels.
SUMMARY
In summary, and to quote G2GBIO Inc., South Korea, who have licensed Micropore’s technology, “The traditional method of manufacturing microspheres faced the challenge of difficulty in achieving uniformity and mass production. There also were limitations, such as lowering the predictability of drug efficacy as a result of low manufacturing reproducibility. The existing microsphere manufacturing method additionally had a problem of difficulty in cleaning and sterilizing, which are essentials for sterile GMP production. Micropore’s microsphere manufacturing technology using membrane equipment overcomes the aforementioned problems. By introducing the newly developed membrane equipment in the GMP manufacturing process, 1 kg of uniform particles can be produced within an hour. This is a mass production scale of about 1,000 vials for Alzheimer’s disease treatment and about 30,000 vials for animal neutering drugs.”9
Together, recent advances in biopolymers and manufacturing technology now enable formulation of injectable drug products to be tailored at will to achieve a target bioavailability in a shorter development time with robust and low cost of manufacture, thereby delivering significantly improved therapeutic outcomes against the target disease.
REFERENCES
- Enas M. Elmowafy, Mattia Tiboni, Mahmoud E. Soliman. Biocompatibility, biodegradation and biomedical applications of poly(lacticacid)/poly(lactic-co-glycolic acid) micro and nanoparticles. J Pharma Invest. 2019;49:347-380. https://doi.org/10.1007/s40005-019-00439-x.
- Makadia HK, Siegel SJ. Poly lactic-co-glycolic acid (PLGA) as biodegradable controlled drug delivery carrier. Polymers. 2011;3:1377-1397.
- Dinesh Dhamecha, Duong Le, Rachel Movsas, Andrea Gonsalves, Jyothi U. Menon. Front porous polymeric microspheres with controllable pore diameters for tissue engineered lung tumor model development. Bioeng Biotechnol. July 10, 2020. doi.org/10.3389/fbioe.2020.00799.
- Farahidah Mohamed, Christopher F. Van Der Walle. Engineering biodegradable polyester particles with specific drug targeting and drug release properties. Published online in Wiley InterScience (www.interscience.wiley.com). DOI 10.1002/jps.21082.
- Dohyun Kim, Tae Hee Han, Seong-Chul Hong, Sun Jae Park, Yong Hak Lee, Hyeongmin Kim, Minwoo Park, Jaehwi Lee. PLGA microspheres with alginate-coated large pores for the formulation of an injectable depot of donepezil hydrochloride. Pharmaceutics. 2020;12:311;doi:10.3390/pharmaceutics12040311.
- Guo-Hua Zhanga, Rui-Xia Houa, Dan-Xia Zhanb, Yang Congb, Ya-Jun Chenga, Jun Fu. Fabrication of hollow porous PLGA microspheres for controlled protein release and promotion of cell compatibility. Chinese Chemical Letters 24. 2013;710-714.
- CPI Report for Micropore Technologies Report Number ESM-0007: Yeast Viability. G Worrall, D Trotter. Centre for Process Innovation. Available on request.
- M Dragosovac, Loughborough University, unpublished report “Production of PLGA particles loaded with leuprolide acetate”. 2012.
- G2GBIO Inc Press Release November 13, 2020. Available on request.
To view this issue and all back issues online, please visit www.drug-dev.com.
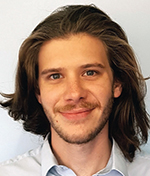
Alex Kerr is a specialist in the field of encapsulation and formulation and heads up Micropore’s formulation development team, investigating a range of techniques relevant to Micropore’s award-winning technology.
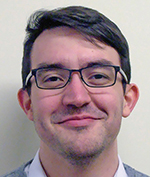
Sam Trotter has a Masters degree in Chemical Engineering and heads up operations for Micropore, overseeing day-to-day lab operations, working with customers, and developing equipment design.
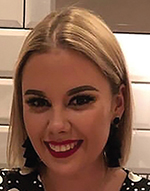
Poppy Maley has a Masters in Clinical Psychology and heads lead generation and customer relations management for Micropore Technologies.
Total Page Views: 7307