Issue:October 2021
DRUG DELIVERY - CAPRO(TM): A New Advance in Polymeric Drug Delivery
ABSTRACT
The estimated global market size of drug delivery products was $1.4 trillion in 2020. Unfortunately, 40% of marketed drugs and 90% of pipeline drugs (mostly small molecules) are poorly soluble in water, which makes parenteral, topical, and oral delivery difficult or impossible. In relation, poor solubility often leads to low drug efficacy. Add in the fact that many other hurdles exist in the form of drug loading, stability, controlled release, toxicity, and absorption – it’s not hard to understand the difficulties in bringing new drug products to market. Additionally, biopharmaceuticals (proteins, peptides, nucleic acids, etc) and combination drug products possess many of these same problematic obstacles that affect efficacy. These challenges, coupled with the complexity and diversity of new pharmaceuticals, have fueled the development of a novel polymeric drug delivery platform called CAPROTM that overcomes a great many bioavailability and delivery obstacles. By leveraging TREKKA Therapeutics proprietary CAPRO polymer platform, pharmaceutical and biopharmaceutical companies can improve dosing accuracy, efficacy, and reproducibility in their drug discovery and drug delivery research. In summary, the CAPRO platform is designed to lower costs, reduce side effects, improve patient compliance, and expand drug access though improved administration methods.
CHALLENGES IN COMPOUNDS & IN DRUG DISCOVERY
The demand for pharmaceutical products worldwide is only going to increase in the coming years, as old and emerging diseases continue to threaten the well-being of people globally. Drug discovery efforts are expected to intensify, generating a large variety of active compounds with vastly different structures and properties. However, it is well known that despite tremendous output of the drug discovery process, the success rate of a candidate compound becoming an approved drug product is extremely low. The majority of candidate compounds are discarded due to various hurdles in formulation and preclinical testing (such as issues with solubility, stability, manufacturing, storage, and bioavailability) before even entering into clinical studies. Therefore, advances in formulation and drug delivery, especially the development of new and versatile biomaterial platforms as effective excipients, may salvage many “difficult,” otherwise triaged, drug compounds, and significantly enhance their chance of becoming viable drug products. Furthermore, breakthroughs in biomaterial platform technologies will also facilitate life cycle management of existing APIs through reformulation, repurposing of existing APIs for new indications, and development of combination products consisting of multiple APIs.
CHALLENGES FACING SMALL-MOLECULE DRUGS
Small-molecule drugs are typically classified by the Biopharmaceutical Classification System (BCS) in terms of their solubility and permeability through biological tissue barriers.1 Of particular challenge are the BCS classes II and IV: both suffer from low aqueous solubility. In fact, 40% of marketed drugs and 90% of pipeline drugs are poorly soluble in water.2 BCS class IV compounds further suffer from low tissue permeability. The absorption of oral drugs takes place in the small intestine, and low solubility limits the maximum drug concentration that can be absorbed. In most cases, slow diffusion is almost always associated with low solubility but can be compounded by small drug surface area and/or slow diffusion rates in the gastrointestinal (GI) tract. The common problem is that a slow diffusion rate may limit drug absorption, particularly when the solubility of the drug product is so low that the drug concentration must be preserved near its maximum solubility limit so that enough drug can be absorbed during the limited time the drug transits the GI tract. Further, the propensity of many drugs to crystallize is another hurdle that makes drug solubilization and absorption difficult.
Current formulation strategies to enhance drug solubility include solubility enhancers (such as cyclodextrin, lipids, surfactants), drug micronization, salt formation, and amorphous solid dispersion in polymeric excipients for oral delivery.3 Organic solvents, though undesirable, are used in some injectables.4 However, these approaches have a number of weaknesses. They are not particularly effective toward highly insoluble drugs and not easily adaptable to achieve various drug-release kinetic profiles. Some formulation processes are complex and require specialized equipment. APIs and excipients may be damaged during melting and extrusion under high temperature. Certain excipients and solvents can be toxic or cause allergic reaction in some patients. Degradation of common polymer excipient families, such as poly(lactide-co-glycolide) (PLGA), produces harmful acidic by-products, causing inflammation.5 PLGA undergoes bulk degradation in vivo by hydrolysis of the ester linkages within the polymer backbone. The reduction of polymer molecular weight and erosion of the polymer measured by mass loss are inconsistent, thus, like other bulk-degrading polymers, it is difficult to control the release kinetics of drugs, especially at high drug loadings. Infiltration of water into the PLGA matrix can also compromise the stability of the drugs during storage and long-term in vivo residence.
CHALLENGES FACING BIOPHARMACEUTICALS
Unlike small-molecule drugs, biopharmaceuticals, including peptides, proteins, nucleic acids (DNA, RNA), are large molecules that present unique challenges.6 A major concern is loss of molecular stability and bioactivity due to degradation by hydrolysis, oxidation, and enzymatic reactions and denaturation due to heat, pH, and organic solvents, resulting in short shelf-life and in vivo half-life. Also, large molecular size and surface charges make it difficult for these biomacromolecules to diffuse across tissue and cellular barriers. Additionally, many peptides and proteins are prone to aggregation at high concentrations, limiting the loading and bioavailability of these drugs.
Bulk-degrading polymers, such as PLGA, are widely used for the controlled release of biological macromolecules, especially peptides and proteins.5 Because most peptides and proteins are hydrophilic, the loading efficiency of these molecules in hydrophobic PLGA is often quite low. The preparation of PLGA formulations requires organic solvents at elevated temperatures and may cause protein denaturation or aggregation. Residual solvent in the drug product can be toxic to the human body. Accumulation of PLGA degradation products results in an acidic environment that can damage and deactivate delicate biopharmaceuticals. Also, the drug release rate is difficult to control, due to the random nature of PLGA degradation and bulk erosion. Hydrophilic polymers, such as polyethylene glycol (PEG), have also been used to facilitate peptide and protein delivery. PEGylation, the conjugation of PEG to peptide and protein drugs, offers protection against enzymatic degradation, aggregation, and non-specific capture and clearance by the reticular-endothelial system (RES).7 PEGylated drugs show longer in vivo half-life and blood circulation time than non-PEGylated forms. However, PEGylation requires complicated and laborious chemical reactions and can be difficult to scale up. Further, hydrogels have been used to encapsulate biopharmaceuticals and provide sustained release; however, the aqueous environment of hydrogels does not protect the cargos from hydrolytic degradation. The release of peptides and proteins from hydrogels usually lasts for days, rather than weeks or months.
CHALLENGES FACING COMBINATION DRUGS
Drug products containing multiple APIs formulated using the same biomaterial excipients present unique challenges in formulations and delivery.8 APIs may have different molecular size, solubility, miscibility, crystallinity, stability, and reactivity. Combining them in the same dosage form and accommodating the disparate needs for solubilization, stabilization, and release profiles will require careful selection of sophisticated solvents and excipients. APIs in combination drugs may act through different synergistic mechanisms to treat diseases. They may have different therapeutic windows (eg, effective and toxic dosing thresholds), different pharmacokinetic profiles (eg, zero order versus first order versus burst release), different duration of release, and different therapeutic targets at the organ, tissue, and cellular levels. The ideal drug delivery system for combination drugs should be able to package multiple APIs together at high doses, maintain their stability, and modulate their spatiotemporal release to reach therapeutic targets.
THE CAPRO POLYMER PLATFORM
TREKKA’s CAPRO polymers provide a flexible and versatile biomaterial platform to address major challenges in drug formulation and delivery. Biodegradable polymers for drug delivery assume a few common physical forms (Figure 1). Hydrophobic polymers, such as PLGA, can form implantable solid matrices or injectable micro/nanoparticles.9 Hydrophilic polymers, such as PEG, can be cross-linked into solvent-saturated gels (hydrogels). In situ forming systems refer to a liquid precursor turning into a solid matrix or gel after injection into the body.10 Such phase transformation is often achieved through molecular assembly or precipitation driven by changes in solvent, temperature, or chemical reactivity. In contrast to these current materials, the CAPRO platform is based on bulk solvent-free polymers in the liquid state (liquid polymers) (Figure 1) – a unique physical state of materials with interesting properties for applications in biomedicine and particularly in drug delivery.
At the molecular level, a typical liquid polymer consists of disentangled chains that are flexible and amorphous with low glass transition (Tg) and melting temperatures (Tm).11 These will ensure that such polymers behave as viscous, Newtonian fluids at room and physiological temperatures. In some cases, disentangled chains of a liquid polymer can be “structured,” forming a solid-like composite with unique rheological properties.12
Only a handful of synthetic liquid polymers have been reported to date for drug delivery applications. Known as “thermoplastic pastes” or “semi-solid polymers,” these materials include polyorthoesters, alkyl polyesters, star PLA-castor oil, and polycarbonates.11,13 Chemical synthesis of such polymers often requires complicated processes that are difficult to scale up. The biodegradation process of polyester-based materials lacks control, and in some cases, leads to the accumulation of acidic by-products. Furthermore, none of these polymers is capable of forming “structured” liquids. The CAPRO polymer platform overcomes these deficiencies and allows for full exploitation of the liquid polymers to address the challenges in drug delivery.
MOLECULAR DESIGN PRINCIPLES OF CAPRO14,15
CAPRO polymers are designed specifically to overcome many of the weaknesses of current polymers for drug delivery. The CAPRO polymers share a generic molecular structure consisting of functional blocks and degradable linkers (Figure 2). The highly modular nature of such design makes it possible to generate a large array of polymers with diverse functionalities. The molecular features of the CAPRO polymers can be engineered to create specific interactions with specific APIs in ways that promote drug dissolution and loading, preserve drug stability during storage, and achieve controlled drug release, leading to improved bioavailability and efficacy.
One class of CAPRO polymers is the CAP-O series (Figure 2). Here, polycaprolactone (PCL) is chosen as the primary functional block for its excellent biocompatibility and low Tg and Tm.16 The linker may contain labile chemical bonds, such as the ortho ester bond, which can determine the kinetics of polymer degradation and in turn, dictate the rate of drug release.17 The length and number of the PCL block (and other potential functional blocks) as well as the chemical structure of the linker can be manipulated independently depending on the need of specific drugs, route of administration, and desirable pharmacological profiles.
ADVANTAGES OF CAPRO POLYMERS IN ACCELERATING DRUG DEVELOPMENT14,15
The CAPRO polymer platform is developed to provide solutions to multiple challenges and hurdles in drug formulation, manufacturing, storage, administration into patients, and in vivo pharmacological action (Figure 3).
APIs
CAPRO polymers are compatible with both small-molecule and large-molecule APIs as well as combination drugs (Figure 4). For poorly soluble small molecules, the CAPRO polymers serve as “macromolecular solvents,” enabling molecular dissolution of the drugs within the polymers. Large molecules, such as peptides, proteins, and nucleic acids, can be incorporated into the CAPRO polymers as particulate dispersion. In relation, they are particularly suited for combination drugs, providing spatially segregated compartments for various APIs within the same dosage form.
Formulation
Formulation using the CAPRO polymers can be very simple. The loading of drugs in the CAPRO polymers can be accomplished by admixing with or without solvent at moderate temperatures. Solvent-free formulation has the advantage of eliminating the concern of residual solvent in the final drug products and any associated toxicity. Moderate temperature during formulation alleviates the potential risk of heat denaturation and decomposition of certain fragile APIs. The “macromolecular solvent,” ie, the CAPRO polymer, can be designed to bind to APIs through multiple sites of molecular interaction. Such cooperative binding imparts greater capacity of solubilization and stabilization of the API, resulting in exceptionally higher loading than what is achieved using simple small molecular solvents.
Scaling-Up & Manufacturing
The synthesis of CAPRO polymers is highly scalable. The CAP-O series is synthesized in three steps. The CAP-A series (containing acetal linkers) is synthesized in a single step. Synthesis can be conducted either in bulk without solvent or with USP class 3 solvents under moderate temperatures. The raw materials used for synthesis are common commercial compounds. We have developed GMP synthesis and purification protocols to manufacture CAP-O series of polymers in 100- to 200-g scale. Manufacturing of CAPRO-formulated drug products can be conducted using conventional admixing devices or standard spray drying apparatus. Furthermore, CAPRO polymers capable of forming structured liquids can be manufactured via 3D printing using either temperature-induced solidification and/or chemical reactive cross-linking methods. Tableting of CAPRO polymers with API is also possible in the presence of appropriate excipients and particulates.
Storage
CAPRO polymers bind to APIs through specific molecular interactions, keeping them solubilized and preventing crystallization during storage. API stabilization can also be augmented by the CAPRO polymers’ capacity of forming structured liquids, which greatly reduces the molecular mobility of the APIs within “gel-like” matrices. Water-sensitive, fragile APIs can be protected from hydrolysis and other forms of degradation by encapsulation within hydrophobic CAPRO polymers, whereas water-soluble biological APIs can be stabilized within hydrophilic or amphiphilic CAPRO polymers.
Administration
The CAPRO polymer platform is designed to adapt to various routes of drug administration. Due to the fact that CAPRO polymers are viscous liquids, CAPRO-formulated drug products can be injected locally into the skin or muscle or ocular sites, to form long-acting depots that release drugs sustainably. In addition, certain CAPRO polymers can form emulsions of micro or nano-size droplets or particles and thus can be injected systemically.18 They also can be applied topically to the skin or other mucosal tissues, such as nasal, buccal, sublingual, rectal, vaginal sites, and enhance the permeation of APIs across these tissue barriers. They can also be adapted to form various oral dosage forms, such as tablets, capsules, gels, and emulsions, and be combined with existing excipients for oral drug delivery.
In Vivo Action
As “macromolecular solvents,” CAPRO polymers can enhance the solubility and stability of poorly soluble drugs, resulting in more efficient absorption and higher bioavailability. The CAPRO polymers can undergo pre-programmed erosion and degradation to achieve either sustained long-term drug release, or modified release that maximizes drug absorption and efficacy. Micro and nano-droplets of CAPRO polymers can facilitate the intracellular uptake of drugs by specific cell types and the intracellular release of drugs within subcellular compartments. Importantly, the CAPRO polymers themselves are constructed from biocompatible building blocks such as PCL and they break down into nontoxic products, which ensures safety for human use.
SUMMARY
TREKKA Therapeutics flexible and versatile CAPRO polymeric drug delivery platform has the potential to enormously impact healthcare. Achieving delivery of poorly soluble drugs and biologics will enhance the efficacy of a wide range of therapeutics for the treatment of a wide range of diseases. CAPRO represents a new and better class of excipients and will provide a new avenue for research and development teams that are dealing with bioavailability and efficacy challenges with new or existing compounds.
REFERENCES
- Rautio, J. et al. Prodrugs: Design and clinical applications. Nat. Rev. Drug Discov. 7, 255–270 (2008).
- Marta Rodriguez-Aller, Davy Guillarme, Jean-Luc Veuthey, R. G. Strategies for formulating and delivering poorly water-soluble drugs. J. Drug Deliv. Sci. Technol. 20, 342–351 (2015).
- Van Hoogevest, P., Liu, X. & Fahr, A. Drug delivery strategies for poorly water-soluble drugs: The industrial perspective. Expert Opin. Drug Deliv. 8, 1481–1500 (2011).
- Thakur, R. R. S., McMillan, H. L. & Jones, D. S. Solvent induced phase inversion-based in situ forming controlled release drug delivery implants. J. Control. Release 176, 8–23 (2014).
- Xu, Y., Kim, C. S., Saylor, D. M. & Koo, D. Polymer degradation and drug delivery in PLGA-based drug–polymer applications: A review of experiments and theories. Journal of Biomedical Materials Research – Part B Applied Biomaterials (2017) doi:10.1002/jbm.b.33648.
- Mitragotri, S., Burke, P. A. & Langer, R. Overcoming the challenges in administering biopharmaceuticals: Formulation and delivery strategies. Nature Reviews Drug Discovery (2014) doi:10.1038/nrd4363.
- Turecek, P. L., Bossard, M. J., Schoetens, F. & Ivens, I. A. PEGylation of Biopharmaceuticals: A Review of Chemistry and Nonclinical Safety Information of Approved Drugs. Journal of Pharmaceutical Sciences (2016) doi:10.1016/j.xphs.2015.11.015.
- Hu, Q., Sun, W., Wang, C. & Gu, Z. Recent advances of cocktail chemotherapy by combination drug delivery systems. Advanced Drug Delivery Reviews (2016) doi:10.1016/j.addr.2015.10.022.
- Fredenberg, S., Wahlgren, M., Reslow, M. & Axelsson, A. The mechanisms of drug release in poly(lactic-co-glycolic acid)-based drug delivery systems – A review. International Journal of Pharmaceutics (2011) doi:10.1016/j.ijpharm.2011.05.049.
- Lin, C. C. & Anseth, K. S. PEG hydrogels for the controlled release of biomolecules in regenerative medicine. Pharmaceutical Research (2009) doi:10.1007/s11095-008-9801-2.
- Amsden, B. G. Liquid, injectable, hydrophobic and biodegradable polymers as drug delivery vehicles. Macromol. Biosci. 10, 825–835 (2010).
- Cassagnau, P. Melt rheology of organoclay and fumed silica nanocomposites. Polymer (2008) doi:10.1016/j.polymer.2007.12.035.
- Packhaeuser, C. B., Schnieders, J., Oster, C. G. & Kissel, T. In situ forming parenteral drug delivery systems: An overview. European Journal of Pharmaceutics and Biopharmaceutics (2004) doi:10.1016/j.ejpb.2004.03.003.
- Wang, C., Wang, W. & Ohlfest, J. R. United States Patent. US10,179,173B2 (2019).
- Wang, W. & Wang, C. United States Patent. US10,226,534B2 (2019).
- Woodruff, M. A. & Hutmacher, D. W. The return of a forgotten polymer – Polycaprolactone in the 21st century. Prog. Polym. Sci. 35, 1217–1256 (2010).
- Tang, R., Palumbo, R. N., Ji, W. & Wang, C. Poly(ortho ester amides): Acid-labile temperature-responsive copolymers for potential biomedical applications. in Biomacromolecules 10, 4, 722–727(2009). doi:10.1021/bm9000475.
- Zhang, L., Zhang, Z., Wang, W., Tabet, A., Hanson, S., Zhang, L., Zhu, D. & Wang, C. Polymer-Based Dual-Responsive Self-Emulsifying Nanodroplets as Potential Carriers for Poorly Soluble Drugs. ACS Applied Bio Mater. (2021) Article ASAP, doi: 10.1021/acsabm.1c00194.
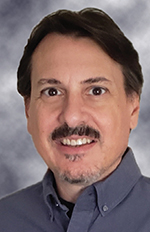
Bob Wieden is the President & CEO of TREKKA Therapeutics, LLC, a spin-off company from the University of Minnesota, that was formed to commercialize and develop advanced polymeric biomaterials to improve the efficacy and safety of drugs. He leads the overall strategy and commercialization efforts to bring this disruptive CAPROTM technology solution to market to improve the patient experience and potentially patient outcomes. He is a growth-oriented business builder that has led a plethora of new ventures for Fortune 500 and private enterprises to fill “gaps” in unmet markets and looks to drive new therapeutic concepts to disrupt existing marketplaces. He can be reached at bobw@trekkatherapeutics.com.
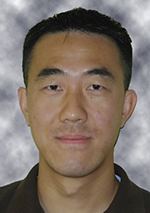
Dr. Chun Wang is an Associate Professor of Biomedical Engineering, University of Minnesota. He is a Co-founder and CSO of TREKKA Therapeutics. He earned his PhD in Bioengineering at the University of Utah and was an NIH postdoctoral fellow at the Massachusetts Institute of Technology. He was a recipient of the National Science Foundation CAREER Award, Wallace H. Coulter Foundation Early Career Translational Research Award, and McKnight Land-Grant Professorship. He served on the editorial boards of the Journal of Controlled Release (2006-2016) and Advanced Drug Delivery Reviews (since 2010). He has published 100 peer-reviewed research articles and reviews and has given over 120 invited talks. His research interest is in polymer-based therapeutic biomaterials with applications in controlled drug delivery, immunotherapy, medical devices, and regenerative medicine.
Total Page Views: 5949