Issue:April 2022
CELL & GENE THERAPY - State of the Industry – Where is C> Headed?
INTRODUCTION
Cell and gene therapies (C>) continue to be at the very center of healthcare innovation and are the fastest-growing areas of therapeutics, having already contributed to some of the most significant disruptions in the pharmaceutical and biotech industries. Continuous advancements in C> are transforming how certain diseases are treated and potentially cured, and significantly changing healthcare outcomes. So, what’s next? Where is C> headed? The following explores the industry dynamics that will shape the C> space throughout the next decade.
COST VERSUS VALUE
The US Food and Drug Administration has approved seven cell and gene therapy drugs, but the new product pipeline has approximately 1,200 experimental therapies, with more than half of these in Phase 2 clinical trials. CAGR estimates are about 15% for cell therapies and nearly 30% for gene therapies, and the Alliance for Regenerative Medicine points to a rapid rise in cell and gene therapy developers, with 1,100 such ventures in 2020, an increase of about 10% from 2019.1-3 The industry is still transitioning out of the early development phase and sits at the very start of the rapid growth phase with a lot of opportunity ahead.
Showing the therapies are providing a lifelong cure and not just another type of ongoing treatment will be key to justifying the pricing and gaining acceptance from patients, providers, and payors, be they insurance or governmental. Unfortunately, when new cell and gene products hit the market, the discussion in media often focuses on the price tag instead of highlighting the availability of a new therapy with the potential to cure instead of simply managing what may have been a previously untreatable disease.
This is creating increased pressure to drive down manufacturing costs – a common challenge across the pharma and biotech industries. In C>, this effort will be focused on several fronts, such as increasing batch sizes, standardizing and streamlining processes, and reducing the cost of the materials used in manufacturing. This will go hand in hand with developing processes that provide high yields of consistent product.
The markets will also have an influence. Where there is a broader market upon which to spread the development costs, such as cancers, where there is usually a larger patient population, therapies can hit a lower price point. For the rare or orphan diseases, it will be harder to spread out costs. But there is potential to achieve lower costs in the long run, as in these cases, it may be fruitful to have a platform, such as a particular viral vector, upon which multiple therapeutics can be more easily developed, thereby reducing some of R&D costs.
VIRAL VECTOR DELIVERY SYSTEMS
The efficacy of a gene therapy depends on the delivery vehicle and its ability to effectively deliver a gene into target cell populations. A variety of delivery vehicles have been employed throughout the years attempting to improve the effectiveness of gene therapy, such as DNA nanoparticles, naked DNA, liposomes (DNA and cationic), polyplexes, and non-viral biological delivery.
However, most clinical trials to date have employed viral vectors for the delivery of genetic material as they are capable of efficiently transducing cells and can achieve long-term expression of the desired genes to deliver sustained therapeutic effects. There are further decisions to be made around the optimal vector platform; the pros and cons of each need to be carefully weighed from a holistic viewpoint of the therapy’s developability, scalability and end-use
Lentivirus (LV)
Lentiviral vectors are best used for therapies in which the patient’s cells are going to be transduced ex vivo. An example would be in delivering a target gene into bone marrow stem cells to treat a disorder originating in the bone marrow. This technique has been used in treating disorders like Severe Combined Immuno Deficiency (SCID), also known as the “bubble boy disease”. This works well for CAR-T therapies in which a patient’s T-cells are transducing and expanded ex vivo before being administered back to the patient. It is simply a difference in the cell type being transduced, T-cells versus bone marrow stem cells.
Lentivectors have an advantage in that they can carry a larger transgene or target gene for delivery, compared to most other viral vector delivery platforms. It is also an integrating virus, so the gene is incorporated into the DNA of the cell and is transferred to progeny cells that originate from the treated cell. Many viral vectors can only enter a cell when the cell is dividing, but this is not the case with LV vectors as they can deliver their transgene into the cell when the cell is “resting”.
The downside is that because LV vectors are large, the immune system recognizes it as foreign. Therefore, it is not a good choice for in vivo use and thus not currently a good option for treating systemic or organ-based disorders. These vectors are still largely produced using transient transfection of 293T cells. There have been several attempts at developing a producer cell line from which clones could be developed, allowing the vector to come from a consistent source for each production. One of the biggest issues is that the VSVG protein envelope used with LV is toxic to the producer cells and thus, these cell lines have met with varying degrees of success.
Retrovirus (RV)
When people talk about retroviruses, they are generally referring to gamma retroviruses – we should note that Lentiviruses are a type of Retrovirus, there are however notable differences both in production and method of action between them. With RV, it is currently feasible to make a producer cell line and develop clones to use for all subsequent productions to give a consistent product from one batch to the next. It is also a large virus capable of delivering a large transgene, so like the LV, it is suited to mainly ex vivo applications. Also, like the LV, it is an integrating virus, however, in the case of RV, they tend to integrate the transgene near oncogenes. When there is a strong promotor on the transgene, it can trigger the oncogene and, in rare cases, this has resulted in patients developing leukemia. So it has largely been replaced by LV vectors with a few applications still using it for treatments such as CAR-T therapies in cancers.
Adenovirus (AV)
The adenovirus has mainly been used for in vivo applications. It cannot carry as large of a transgene as the retro- and lentiviruses. The most notable problem is that most people have been exposed to an AV at some point in their lifetime (common cold), and the immune system recognizes it and puts up a defense before transfection can take place in most cases. It should be noted though there has been work done using Adenoviruses as the viral vector platform for vaccines such as the J&J Covid-19 vaccine.
Adeno-Associated Virus (AAV)
Throughout the past few years, AAV has been the shining star in C>. It is small so it doesn’t carry as large a transgene. However, its size means it can often slip past the immune system without eliciting a major response, at least when first used. This makes it ideal for in vivo treatments in specific organs or for systemic disorders. The downside is that it is not an integrating virus. The plasmid that is delivered generally stays free of the cellular DNA and thus is not passed on to the cell’s progeny. In theory, over time, the therapeutic benefits decrease, it remains to be seen just how much of an impact this has over the life of a patient. Some have suggested that subsequent rounds of treatment could be done; however, some attempts have resulted in significant immune responses – time will tell if longevity of the therapy is really an issue. One other item that has been seen, at least in the laboratory, is that over time, some of the transgene plasmids do end up integrating into the cellular DNA. The problem is that much like the RVs, they seem to be integrating near oncogenes. Again, it remains to be seen if this is an issue long-term or if it is just unique to the animal model and the laboratory setting.
WHAT’S NEXT?
Throughout the past 5 or 6 years, a lot of the excitement, especially for investors, has been around cancer therapies. That work has largely been on bloodborne cancers, but there are now approaches being applied to solid tumor therapies. There is likely to be a continued focus on LV and it being used in other CAR-T and Natural Killer cell therapies both for bloodborne cancers as well as solid tumors.
As previously noted, most LV vectors currently use the VSVG envelope, and one area of innovation will involve developing other envelopes to see if there are ways to use LV vectors in vivo and targeting specific organs or used in combination with some immune suppression to reduce the immune response to the virus.
Other platforms will likely develop over time. There has been interest in foamy viruses – a RV belonging to the Spumavirus genus – but they proved extremely difficult to make. Recently, there has been a great deal of interest in using bocavirus as a vector platform, it shows promise in systemic applications like an AAV, but with the added benefit of being capable of carrying a transgene almost as large as that of an LV vector. However, innovation in the space must be facilitated through investment and addressing several challenges that are currently hamstringing progress.
CHALLENGES AROUND CAPACITY, TECHNICAL CAPABILITIES & EXPERTISE
Physical capacity to produce gene and cell therapy products continues to be a bottleneck. Even as more capacity is added, the industry seems to be growing even faster. Some of the expansion is being hampered by the lack of availability of materials – the supply of items, such as plasmids, media, and the numerous disposables from pipettes to bioreactors, has continued to be tight.
The solution will, in part, come from increasing the production of these materials. There also has to be a greater focus on learning how to better utilize the supply so more product can be manufactured with the same amount of material.
The ability to scale up the manufacture of therapies in a cost-effective manner is also essential. The industry must find systems that can be run as a “small” version for the preclinical and early trial work but then be scaled up into larger equipment for commercial use, rather than simply scaling volumes by duplicating small systems that inherently uses more resources and physical space within facilities.
The availability of a workforce with appropriate skills is also going to continue to be a challenge. Currently, the industry is largely clustered in a few locations – in the US, there are a few key metropolitan areas on the east and west coasts that have very high concentrations of C> companies. As a result, the companies in these locations are running into problems finding the workers they need. This competition for the limited number of workers is driving up wages and that is anathema to the idea of reducing costs. It also means projects are delayed because the expertise and workers needed to do the work are not available. There may be several approaches to solving this. The industry may expand into locations other than the east and west coasts; a longer-term solution however would involve federal, state, and local governments supporting and funding dedicated life science workforces. The cities, states, and countries that invest in educating and training a workforce will attract the companies and the jobs and tax dollars they bring. Any country that wants to stay in the forefront of this industry must be able to supply the workers, otherwise they will see the industry and jobs move elsewhere.
REGULATORY APPROACHES
A decade ago, the new treatments in the G&CT space advancing to the clinic sparked excitement about the eventual path to commercialization. This spurred regulatory agencies to work with researchers and companies to move these treatments forward, but given the uncharted waters, even the questions to be asked were evolving. Now, some of these treatments have been approved, and parties on both sides of the regulatory equation have learned how to evaluate the development phase and the important regulatory factors and questions. For example, progress in regulatory perspectives has led to a preference for a single producer cell line clone for use through all development phases.
Regulators, such as the FDA and the European Medicines Agency (EMA), have published formal and informal guidelines to help viral vector companies produce products. Due to the evolving nature of the C> industry, regulations surrounding these products are updated to keep up with the rapidly growing field and its development – for example, the FDA has provided or updated nine guidance documents within the last 2 years.4
This will continue to evolve, and each new type of therapy will bring its own learning curve to the regulatory process. Moreover, it will continue to be important for gene therapy developers and manufacturers to work hand in hand with regulatory agencies as more open discussions around issues, challenges, and best practice will put the industry in a better position to collaborate on any future regulations and guidance.
DRIVING THE INDUSTRY FORWARD
If the industry wants to keep moving up the steep growth curve, then capacity and raw materials must be readily available. The innovations in therapies, platforms, and processes will all come with time, money and increasing availability of resources.
Investment into basic research at the university level and into small start-ups coming from venture capital, private equity, industry, and the capital markets will be essential – university labs and small start-ups are well-positioned for the needed innovation. Invariably, these companies start out with a small team that is willing to take risks and then grow into larger companies or go through a series of acquisitions that bring those innovations into larger biotechs and big pharma. These larger entities can use their resources and expertise to commercialize the product and get it to market.
Outsourcing to niche CDMOs with the smaller scale capabilities and expertise required to develop scalable processes at this stage will be essential to expediting a C>’s path to market.
REFERENCES
- https://www.grandviewresearch.com/industry-analysis/cell-therapy-market
- https://www.marketsandmarkets.com/ Market-Reports/gene-therapy-market-122857962.html?gclid=CjwKCAjw6qqDBhBEiwACBs6x4bpV22MV4VlZTpbRoGbtjlA0vbzRECcCcSWb64ZQrIoZ8hoA6e9cxoCUsQQAvD_BwE
- http://alliancerm.org/wp-content/uploads/2021/03/ARM_AR2020_FINAL-PDF.pdf
- https://www.fda.gov/vaccines-blood-biologics/biologics-guidances/cellular-gene-therapy-guidances
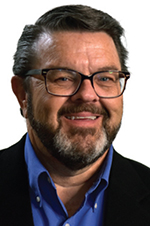
Bill Vincent, following 25 years in the pharma sector, set out to bring academia and pharma closer together by founding Genezen in 2014. Initially focusing on contract manufacturing, Genezen quickly expanded to include cell manufacturing and testing services. Today, as Executive Chairman, he focuses on business strategy, whether that is evaluating new opportunities and technologies, or facilitating activities for Genezen’s clients and employees. Prior to Genezen, he was Founder and President at Rimedion (acquired by Ossium Health in 2017).
Total Page Views: 6791