Issue:March/April 2025
CELL-FREE GENE SYNTHESIS - Eliminating Critical Bottlenecks in Genetic Medicine Development
INTRODUCTION
The rising tide of genetic medicines is shifting the role of synthetic DNA in pharmaceutical development. Custom DNA constructs have been used in research since the inception of advanced molecular biology techniques, but with the clinical success of genetic medicines, DNA is now often a critical precursor to the active pharmaceutical ingredient (API), or part of the pharmacologic substance itself. This article explores the market trends, challenges, and opportunities new DNA production technologies provide to accelerate genetic advances in healthcare.
GROWING DEMAND FOR DNA
The recent growth in the biopharmaceutical arena can be described as nothing short of explosive. In 2022 alone, the industry witnessed a phenomenal acceleration, surging to 333.09 billion USD with a trajectory to reach 856.1 billion USD by 2030.1
Traditionally propelled by monoclonal antibodies and other biologics, the market dynamics are undergoing a major shift. Genetic medicines, comprising cell and gene therapies as well as RNA-based vaccines and therapies, have emerged as major actors poised to claim a substantial share of the market. This shift, likely intensified by the growing demand for personalized medicine and precision therapies as well as the global impact of the COVID-19 pandemic, has put an even bigger spotlight on the need to accelerate the pace of discovery and scale-up for clinical testing. In response to this demand, cell-free DNA synthesis and cloning technologies have stepped into the limelight.
GENETIC MEDICINES: KEEN CLINICAL INTEREST BUT AN APPROVALS BOTTLENECK
Despite the ambitious growth predictions, the number of genetic medicines approved by the Food and Drug Administration (FDA) remains relatively low. The number of FDA-approved cell and gene therapies increased from five in 2022 to just seven in 2023. RNA therapy approvals increased from five in 2022 to eight in 2023. Many candidates continue to fail clinical trials due to low efficacy or adverse effects.
Regardless of the low FDA approval rates observed thus far for genetic medicines, the entire field is expanding rapidly as technologies like AAV, lentiviral, and other vector platforms for both in vivo and ex vivo genetic modification of target cells are translated into therapies. Applications for chimeric antigen receptor-modified T-cells (CAR-T) are also growing quickly, primarily in oncology but also targeting autoimmune and other diseases. A search of the NIH clinical trials database yields almost 2,500 studies that are currently recruiting participants for gene therapy and over 700 currently recruiting for CAR-T trials.2
The transition of DNA to a biopharmaceutical construct is leading to increasing scrutiny and guidance from the FDA3 and EMA,4 with efforts to harmonize the regulatory frameworks for the manufacture of genetic medicines. DNA constructs intended to be used in vivo in human beings require GMP manufacture.5 However, DNA utilized as a template for RNA (or mRNA) transcription may be defined as a starting material rather than API.6 In contrast, DNA used to modify cells ex vivo, with the cells delivered as the therapy, might be considered as a precursor or an API.
INHERENT RISKS OF CELL-BASED DNA APPROACHES
Cell-based cloning is currently the industry-standard method to isolate, purify, and scale the production of custom DNA for the development of most genetic medicines. However, using this decades-old method to assemble, identify, and amplify sequence-perfect DNA constructs has significant drawbacks:
Time & Cost: Time and cost are always primary considerations and drivers at the center of drug development, and genetic medicines are no exception. From ordering oligos to sequence assembly, cloning, and purification, the hierarchical build cycle to attain long, sequence-perfect DNA takes ample time as well as dedicated equipment and highly-trained personnel — all costs that significantly add to the overall expense of development. These costs may manifest as dedicated internally resourced biofoundry labs or outsourced CROs/CDMOs.
Additionally, promising DNA candidates can have highly complex sequences that take longer to produce and thus bear a cost in go/no-go decisions of moving a nucleic acid-based drug forward. Viable candidates and targets can be abandoned prematurely due to time and cost considerations.
Failures in Scaleup: There are instances where DNA assemblies are toxic to the host cell or are structurally challenging to assemble, resulting in cloning failure or mutations in the intended assembly. These kinds of failures are why GMP production of plasmid DNA requires a master cell bank: both the plasmid and host strain may require significant engineering to result in a construct that will amplify the DNA stably. Such genetic engineering often costs several hundred thousand dollars and takes several months. In contrast, cell-free DNA production approaches do not rely on host cell-mediated replication and therefore do not exhibit these kinds of failures.
Risks of Vector and Bacterial Origin DNA: For plasmid-based approaches, bacterially derived selectable marker and replication of origin DNA sequences are part of the isolated plasmid DNA and can pose downstream risks for both horizontal gene transfer and virus-mediated gene therapy approaches.7 For example, AAV-mediated gene therapy approaches that relied on plasmid constructs produced between 3-26% viral particles containing an antibiotic resistance gene of plasmid backbone origin. The percentage of AAV particles that contain incorrect sequences can vary significantly depending on the production method; in fact 10-90% of AAV particles may be “empty” or incorrectly filled, the exact proportion dependent on the efficiency of the packaging process and the QC measures implemented during production.8 Obviously, the integration of plasmid-originating DNA into the genome of transduced cells is a serious safety concern for AAV gene therapy.9 These risks can be reduced using small backbone and antibiotic-free selection methods such as minicircle and Nanoplasmid (Aldevron) approaches, which reduce the size and nature of the DNA elements required for DNA amplification. However, the safest approach is to eliminate bacterially derived sequences entirely.
Contaminants & Testing Burden: Cell-based DNAs typically contain contaminants including bacterial genomic DNA, RNA, protein, and endotoxin (e.g. LPS) and require additional time and costs for ensuring complete removal. Endotoxin is the most critical risk factor, since contamination levels can confound downstream cell-based assays, and removal of endotoxin must be confirmed prior to clinical use. The most widely accepted and sensitive assay used for endotoxin testing, the limulus amebocyte lysate (LAL) assay, represents yet additional cost and instrumentation. The removal of contaminating RNA, DNA, protein, and endotoxin from cell-based DNAs adds significantly to production cost and time and increases the risks associated with a failure of any one of these purification steps.
Supply Chain Risk: Many small- and mid-sized biotech and biopharmaceutical companies outsource the scaleup and production of complex therapies like gene therapies. These third-party organizations offer specialized expertise, advanced manufacturing capabilities, and scalability, allowing companies to focus on core research. However, using a CMO or CDMO for cell and gene therapy development and/or manufacturing introduces several supply chain risks. These risks include potential delays in production due to the availability of an external CMO/CDMO, their capacity constraints, and obligations or prioritization of other clients’ projects. Outsourcing to external partners also could expose intellectual property and complicate regulatory compliance. Furthermore, any disruption in the CMO/CDMO’s operations can lead to delays in clinical trials or product launches, ultimately affecting patient access and market competitiveness.
CELL-FREE CLONING: A DE-RISKED PATH TO DNA PRODUCTION
Forward-thinking biopharma companies seeking ways to produce high-quality therapies with greater speed and efficiency are already exploring the use of new DNA production methods including cell-free DNA cloning to accelerate pipelines, improve the purity and fidelity of DNA constructs, and make their overall DNA-based drug development operations less costly, error-prone, and brittle.
Cell-free DNA cloning is advancing rapidly. Rather than performing laborious cloning of imperfect DNA fragments in bacteria, cell-free cloning enables researchers to shift the effort normally spent building DNA to analyzing downstream results, improving designs, and exploring a broader sequence space instead. Today, researchers can receive 1-60 μg of sequence-perfect, high-complexity, NGS-verified dsDNA molecules up to 7,000 bases long within 6-8 business days — manufactured cell-free. Such cell-free DNA production technologies are just starting to gain a foothold, and the speed, quality, and length of the DNA produced are improving. For example, cell-free DNA manufacturing companies like Elegen are already delivering high-complexity, clonal-quality DNA at lengths up to 15 kb in as fast as 10 days to early access program customers.
The benefits of cell-free cloning extend well beyond speed. Cell-free cloning can handle a wider range of complexity more reliably than plasmids, thus improving the chances of success. Furthermore, since the DNA produced never touches a bacterial cell, the risk of endotoxin contamination (and other bacterially derived contamination) is eliminated. Finally, cell-free cloning successfully enables the synthesis of sequences that fail to propagate through conventional methods using bacterial cell cloning.
NOT ALL “CELL-FREE” APPROACHES INVOLVE CELL-FREE CLONING
In recent years, emerging DNA suppliers have featured technologies that enable the production of large quantities of DNA without using bacterial cells. Since these technologies avoid the time and high costs of growing and maintaining bacterial colonies in large fermentation tanks, they present an attractive option for biopharma.
These “cell-free” technologies use isothermal enzymatic amplification techniques such as rolling circle amplification (RCA) to produce amplified copies of a circular DNA template. While promising as a replacement for the conventional replication of DNA through the propagation of bacteria, the circular template is typically produced by cell-based cloning, bringing all the baggage associated with cell-based cloning into the production workflow.
To avoid generating replicates of an imperfect sequence with RCA, weeks of time and effort are required to produce a sequence-perfect template for amplification. This template also needs to be purified of contaminants, adding more time and expense to the overall process.
NEXT-GEN DNA: CLINICAL-GRADE, CELL-FREE DNA PRODUCTION IS THE FUTURE
With new technologies emerging to offer cell-free cloning and cell-free amplification of synthetic DNA, it’s easy to envision a state where genetic medicines are more efficiently developed and produced. By eliminating the drawbacks of cell-based approaches, cell-free DNA production saves several weeks in the discovery phase and many months in the scale-up phase for clinical testing.
For personalized genetic medicines, the cost of growing and maintaining a master cell bank to produce DNA for each patient is prohibitive. With personalized RNA neoantigen vaccines entering clinical testing, it’s only a matter of time before personalized genetic medicine makes the transition from clinical investigation to routine therapeutic – provided the method of DNA production is sequence-perfect, scalable, and cost-effective.
Thanks to a handful of innovative DNA manufacturers, the era of next-gen DNA manufacturing, produced entirely cell-free from digital design to end product, at any length and complexity, and ready for use without further manipulation, is very near. In the race to produce the next wave of advanced genetic vaccines, cancer therapeutics, and personalized therapies, cell-free DNA production is the one asset biopharma cannot afford to overlook.
REFERENCES
- https://www.biospace.com/article/biopharmaceuticals-market-size-to-hold-usd-856-1-bn-by-2030/
- https://www.clinicaltrials.gov/.
- https://www.fda.gov/media/113760.
- https://www.ema.europa.eu/en/documents/scientific-guideline/guideline-quality-non-clinical-and-clinical-aspects-gene-therapy-medicinal-products_en.pdf.
- https://www.fda.gov/media/113760.
- https://ispe.org/pharmaceutical-engineering/ispeak/plasmids-and-their-role-mrna-technology.
- https://www.ncbi.nlm.nih.gov/pmc/ articles/PMC10280095/.
- https://doi.org/10.1371/journal.pone.0269139.
- https://pubmed.ncbi.nlm.nih.gov/28131313/.
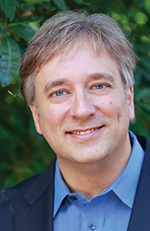
Marc Unger is the Chief Scientific Officer at Elegen, with more than 20 years of expertise bringing pioneering technology and products to the biotechnology and life sciences industries. After a successful career as CSO of Fluidigm, whose products and technology were based on the Multilayer Soft Lithography technology he invented, Marc took on the challenge of transforming the DNA supply market, using a combination of microfluidics and advanced molecular biology to deliver high-throughput, NGS-verified, cell-free gene synthesis at industry-leading length, complexity, and speed. At Elegen, Marc is responsible for overseeing research and product development. He is driven by Elegen’s mission to solve the DNA Write challenge, enabling programmable biology to drive advancements in genetic medicine, agriculture, and synthetic biology.
Total Page Views: 1304