Issue:January/February 2021
BIOSIMILAR DEVELOPMENT - Biosimilars: The Process & Quality System Approach to Clinical Applications
INTRODUCTION
Biosimilars are medicines that are highly similar to their approved reference biologics as they claim to have no clinical differences in purity, potency, and safety. For regulatory approval for a biosimilar in the US, a sponsor must demonstrate that its product is highly comparable to an FDA-approved biologic, and that any residual differences do not affect the biosimilar’s safety and effectiveness. The sponsor’s claim plays a pivotal role for use of biosimilars in specialty therapy categories, such as immunology, endocrinology, and oncology. The new discoveries of innovative biosimilar products continues to challenge the clinical treatments for patients suffering from chronic diseases (ie, carcinoma, sarcoma, lymphoma, etc). These new innovative treatments have placed immense economic burden on emerging market developments and healthcare systems delivery. According to the European Medicines Agency (EMA), there are assessment reports showing a decrease in costs and marketing of biosimilars, leading to ease of access for patients. The following addresses biosimilar developments and future innovations. Emphasis is placed on quality system approaches to the development and availability of new biosimilar products. For approvals of new biosimilars, the sponsors of premarket applications must present analytical and biological characterization to demonstrate that a proposed biosimilar is highly similar to a licensed reference product. The premarket application protocol requires a sponsor to describe the biosimilar product’s PK/PD clinical data comparing its safety, efficacy, and immunogenicity to that of the reference product. Emphasis is placed on design of studies, extrapolations, interchangeability, and c-GMP risk-based monitoring criteria. A brief description is presented on risk-benefit analysis that guides the clinical use of the new biosimilar drug product by providing patients’ organized data and appropriate labeling information in conformance with the new biosimilar drug’s intended clinical use.1,2
DEVELOPMENT STRATEGIES FOR BIOSIMILAR PRODUCTS
Biosimilar medicines have a profound impact on patients suffering from many debilitating and life-threatening diseases, such as rheumatoid arthritis, cardiac myopathies, leukemias, lymphomas, multiple sclerosis, and various oncogenic cancers. Biosimilars are copies of biological medicines and require stringent comparison against their licensed reference products (design controls, CMC, GMP, nonclinical, and clinical). Biosimilars share the same amino acid sequences as their comparative biologics, but may consist of proteins having post-translational changes due to manufacturing processes (ie, glycosylation, phosphorylation, etc) These types of modifications may impact immunogenicity. The impact of post-translational changes require similarity studies, such as analytical/biological, nonclinical, and clinical in order to ensure the safety and efficacy profiles of the resulting new biosimilars. Thus, developing new biosimilars require robust strategies to achieve the goal of reduced development costs. From GMP perspectives, Chemistry, Manufacturing, and Controls (CMC) will require comprehensive comparison data requirements along with nonclinical and clinical studies. When the final data and information are available, then a global strategic roadmap can be constructed to pursue the ultimate goal of providing quality biosimilars for patients in need of treatments for debilitating and life-threatening diseases. For clinical assessment, the comparative PK and/or PD data in addition to comparative immunogenicity, safety, and efficacy data become essential for evaluation purposes. However, the PD measures should be relevant to clinical outcomes after dosing to ascertain PD response in terms of sensitivity and specificity to detect clinically meaningful differences. When all of the aforementioned essential elements are addressed, a strategy can be developed with regard to manufacturing process development, biosimilarity testing, scale-up, nonclinical testing, clinical studies, marketing, and clinical utility outcomes.1-8
CLINICAL ASPECTS OF BIOSIMILAR DRUGS EVALUATION
Biosimilar medicines have been essential in the treatment of diseases ranging from autoimmune diseases to various types of cancers. The US biosimilar approval process requires a thorough characterization of the molecular structure of the proposed biosimilar product with clinically meaningful outcome. In other words, the proposed biosimilar is expected to produce clinical outcomes that are not significantly different from those expected with licensed reference biologic drug approved by the FDA. This publication is intended to present guidances in reference to FDA’s regulatory framework for organizing sponsor’s data in biosimilar 351(k) application. An example is the anti-CD 20 monoclonal antibody, rituximab, which has revolutionized the treatment of patients suffering from non-Hodgkin’s lymphoma (NHL). In 2014, the National Cancer Institute (NCI) stated that since 1997, deaths due to NHL decreased each year and continue to fall. Recent information in regard to immune-oncology therapies have provided new treatment examples for patients with advanced cancers, such as lung cancer and melanoma. Other biologic medicines, such as epoetins, infliximab, and filgrastims have played important roles in the treatment of patients with serious life-threatening situations. These types of biologic medicines were developed based on data presented in comparison to approved reference products. These developmental paradigms have the potential to improve the affordability and accessibility and cost of biosimilar medicines (ie, TNFa inhibitors known as Humira, Enbrel, and Remicade). These studies have provided opportunities for developers and providers to make it available to patients and payers potentially significant cost savings. Neverthless, these kinds of advancements and opportunities make it possible for patients with clinical outcomes that are meaningful in comparison to original biologic-innovator drug products.9,11
DEVELOPMENT PERSPECTIVES
The developmental perspectives consist of characterization of basic structures, such as protein backbone (physicochemical) properties of the biosimilar product and biological activities associated with it. It may not be limited to primary, secondary, tertiary, or quaternary protein structures, but post-translational modifications may occur due to manufacturing processes, which may influence the functionality of the protein. For instance, effector mechanisms associated with monoclonal antibodies may require assessing the biological activity of the biosimilar product in terms of receptor binding and pharmacodynamic effect. Finally, other properties of the biosimilar poduct, such as formation of residual aggregates may require testing and acceptability.8
Sponsors of innovative new biosimilar drugs follow the appropriate ICH guidance in regard to preclinical characterization of safety and effectiveness.4,6,9 These studies involve testing in a relevant animal species, which represent appropriate receptor binding studies. The developmental paradigm for a new biosimilar drug emphasizes clinical evidence to demonstrate that safety and efficacy of each indication for use is similar to the reference product’s clinical safety and efficacy information. While development of innovative biosimilars usually focuses heavily on clinical studies, the FDA’s guidances describe similarity studies at the physicochemical and biological level using a variety of analytical techniques (ie, cellular bioassays are considered to be useful in comparing receptor binding kinetics and bioactivity).8
CLINICAL STUDIES
The sponsors of biosimilar 351(k) applications have shown that clinical studies usually include side-by-side comparisons of pharmacokinetics, pharmacodynamics, immunogenicity, safety, and efficacy as described below:
Pharmacokinetic Studies: The pharmaco kinetic (PK) profiles of biosimilar biologic drugs are dependent on many factors, including product-specific characteristics, such as small differences in the quality attributes of a candidate biosimilar in comparison to a reference product, which may potentially lead to differences in drug absorption, distribution, metabolism, or excretion.11 These types of clinical studies (ie, human PK/PD studies) play a central role in the biosimilar developmental process. Furthermore, these studies provide sensitive tools to assess potentially clinically relevant differences between candidate biosimilar and reference biologic. Human PK studies are generally conducted in a well-defined healthy-sensitive population who are not prescribed other medicines that could interfere with baseline human PK studies.8,12
Clinical immunogenicity studies in a healthy-sensitive population also provides information in regard to duration studies (antibody titers) after extended exposure to biosimilar product.8,9 Confirmatory safety and efficacy studies are helpful in assessing clinically relevant differences between the candidate biosimilar and reference product. These types of studies depend on whether PK studies are designed and conducted in settings to detect sensitivity to change. The type of study performed depends on whether that study is designed for parallel or cross-over groups. Generally cross-over studies are not feasible due to the long half-lives associated with many biologic products, particularly monoclonal antibodies. For biosimilars with relatively short half-lives, such as filgrastims, insulin, or certain fusion proteins, cross-over studies are preferred. Additionally, host factors, such as receptor affinity and patient status, may affect the disposition and clearance of biosimilar. Furthermore, concomitant medications (ie, immunosuppressants) may affect the PK of the biosimilar products and could mask differences between the candidate biosimilar and the reference product. For those situations, particularly where monoclonal antibodies are used for cancer treatment, patients receiving first-line therapy with minimum heterogeneity/prior therapies may show minimum impact on the clinical PK profile of candidate biosimilar and reference product.8,11
It is important to consider the design of study for biosimilar’s route of administration and absorption kinetics for comparative PK profiles. Bioequivalent testing protocols are very helpful to assess PK similarities. Many biologics biosimilars are usually administered via intravenous injection or infusion, making bioavailability approximately 100% possible. However, for a candidate biosimilar intended to be administered subcutaneously, simply comparing Cmax and AUC methods may not be suitable to assess the PK similarity of the candidate biosimilar and its reference. In those situations where there are differences in absorption and distribution modes, analysis and comparison of additional PK parameters (ie,T1/2, Ke, and Cl) distribution and clearance of biologics biosimilars may be useful. From a clinical perspective, methods used to determine serum concentrations in test results from volunteers/patients need to be validated with the guidelines and standards based on (National Committee for Clinical Laboratory Standards-NCCLS). Notably, NCCLS recognized ligand-binding assays are used for the detection of patient sample analytes for US FDA premarket approvals.1-14
Pharmacodynamic Studies: Human pharmacodynamic (PD) profiles play a central role in detecting any clinically relevant differences that may exist relating to the assessment of biosimilarity between a biosimilar and reference biologic.13 PD testing is aimed at determining a safe dose range in which a biosimilar drug can be administered and the methods of absorption and distribution in the body are determined. A primary consideration in these studies is limiting risk to the subjects and determining safety or toxicity limits. These studies usually include PK and PD testing to help establish the relationship between biosimilar drug dose and plasma concentration levels, as well as therapeutic or toxic effects.8,12,13
Clinical Safety & Efficacy Studies: Clinical safety assessment for biosimilar products consist of a comparison of the overall adverse event profile inclusive of specific types of adverse drug reactions occurring after the initiation of treatment. It is useful to compare the types of hazards and severity levels of adverse reactions in those events that have been observed throughout the reference product’s life-cycle in order to determine whether the candidate biosimilar product has shown new safety concerns. This type of study helps selecting a patient population that determines likelihood of detecting a difference in critical control points in the assessment of clinical differences. This may become an issue for the assessment of safety profile parameters for testing the biosimilar product’s side-by-side comparison for monotherapy versus concomitant therapies. This type of testing in a relatively homogeneous population may increase the ability to detect differences in safety parameters by reducing perplexity that may occur due to the use of concomitant medications and/or presence of concomitant conditions. This type of testing is helpful in detecting meaningful differences between safety profiles of biosimilar assessment. For this perspective, appropriate risk management study design and post-marketing surveillance for new biosimilars are crucial for the strengthening of the safety database. Therefore, this type of approach, for the proposed labeling of the biosimilar product indicates the same risks to patients as the reference product’s labeling.
Clinical efficacy assessment for a biosimilar product is a key component of the FDA’s approval process. When designing the clinical efficacy studies, it is important to consider the relevant mechanism(s) of action considering all the indications for use sought for approval. It is known that some biologics can function through multiple mechanisms of action, and the mechanisms involved in the treatment of one disease may not be the same as mechanisms involved in the treatment of other diseases. The ability to detect a difference is of utmost importance for the candidate biosimilar’s development. In order to maximize the sensitivity of a clinical efficacy study, sponsors should perform a thorough review of the available clinical data for the reference product in order to determine the population-endpoint associated with the study database. By performing a thorough systematic review of data available for the reference product, the biosimilar product’s sponsor can identify critical control points of the new biosimilar product in terms of magnitude of effect and the timing of response that are necessary to guide study design in establishing clinically meaningful similarity margins. The primary endpoints should provide adequate sensitivity margins to detect differences in efficacy of the candidate biosimilar in comparison to the reference product. The clinical sensitivity protocol (ie, ability to detect the endpoint differences) is important for the candidate biosimilars. In order to achieve the maximum clinical efficacy sensitivity, the protocol should include both selection of well-defined populations and endpoints that in combination will be sensitive to detect differences that may provide clinical efficacy profile of candidate biosimilar in comparison to the licensed reference product. This type of data comparison provides assessment of candidate biosimilar’s population endpoints that may be associated with a large effect size and a robust historical reference product’s available dataset. These studies performed in comparison to reference product, the sponsor of candidate biosimilar 351(k) application can identify manufacturing critical control points inclusive of the magnitude of effect and the timing of response that are necessary to establish clinically meaningful similarity margins. The primary goal for determining endpoint(s) should be to provide acceptable sensitivity to detect differences/similarities in clinical efficacies of the comparative data. For instance, there are several endpoints commonly used to assess the efficacy of biosimilar products.15,16
Generally, overall survival is considered a quality indicator for the demonstration of clinical efficacy for innovative new biosimilars for oncology treatments. Comparing endpoints for early applications, such as response rates or progression-free survival may be more appropriate in some oncology settings. The important factors being the overall affect of study population endpoints and the timing of therapeutic effects in regard to duration of treatment and follow-up. The comparative studies to demonstrate clinical efficacy of new biosimilars should be designed and powered to test a hypothesis of equivalence, and this should include randomization and double-blinded factors. The selection of equivalence margins should be part of predesigned protocols based on statistical applications and historical data available for the reference product. Predefined equivalency margins include differential criteria for efficacy determination considered clinically meaningful.17
Immunogenicity: Immunogenic studies have an impact on PK/PD, safety, and efficacy of biosimilars. Structural and manufacturing changes in a biosimilar can have different immunogenic responses. For instance, formulation changes in a product containing epoetin alfa have been reported to show a significant rise in the number of cases of red cell aplasia in chronic kidney disease patients due to generation of neutralizing antibodies that cross-reacted with endogenous proteins.18 The formation of anti-drug antibodies (ADA) has been reported with severe acute infusion reactions affecting immunogenicity responses in patients.19 Furthermore, anti-drug antibodies (ADAs) have been shown to interfere with the clinical efficacy of biologic drugs, such as the anti-TNF antibodies that are useful in the treatment of a number of autoimmune diseases.20 The modified complexity of biosimilar structures has been reported that differences in post-translational modifications, such as folding and conformational changes, could lead to differences in the elicit response to immunogenicity.21,22 Therefore, it is essential that sponsors of new biosimilar applicants assess the formation of ADAs in comparative clinical studies in order to determine whether processed molecular differences might lead to differences in the immune responses, which subsequently could affect safety/efficacy of the new biosimilar product.
In designing immunogenicity studies, patient-specific factors play a key role in the interpretation of data (ie, genetic factors, age, concomitant medications, duration, and route of administration, previous exposure to similar products – any or all of these factors may contribute to patient’s risk of developing ADA against the candidate biosimilar). Also the underlying diseases of study participants may influence the rate of ADA against a particular biosimilar product. It has been reported that infliximab had shown rate changes from 7% to 61% in patients with psoriasis, ankylosing spondylitis, Crohn’s disease, and rheumatoid arthritis.14,23 All of these factors should be considered in design and interpretation of the immunogenicity studies for the new biosimilar product.24
Extrapolation: The concept for extrapolation is based on the principle that biosimilar product has demonstrated that intended clinical use and its outcome will not differ meaningfully whether a patient receives the candidate biosimilar or its reference product. Extrapolation must be supported by scientific evidence of the candidate biosimilar’s human PK/PD, safety, and efficacy data in a well-defined patient population based on a similar safety and efficacy profile as reference biologic. It is essential that both the candidate biosimilar and licensed reference product share the same mechanism of action.10 The biosimilar sponsor need not conduct clinical studies in every indication for use described in the reference product’s labeling. Instead, the FDA guidance advises the biosimilar applicant to conduct clinical evaluations in one or two indications, then provide scientific justification for extrapolating clinical data to support a determination of biosimilarity for each condition of use for which licensure is sought. The underlying rationale under the concept of extrapolation is the scientific principle that biosimilar protein structure determines the molecular (output) function, clinical PK/PD data, safety, and efficacy outcome of the proposed biosimilar product.
The essential biosimilar parameters of extrapolation are listed below:
- The uncertainty margin or acceptable analytical/functional differences between the candidate biosimilar and the licensed reference product
- The mechanism of action (MOA) of each indication for use and the justification that the residual differences will not contribute to any meaningful differences in the clinical safety and efficacy of the biosimilar’s intended use sought by extrapolation
- High similarity in PK/PD comparisons of the candidate biosimilar and the reference product’s established indications for use and the justification that any residual differences will not contribute to misinterpretation of data (extrapolation justification that residual differences will not lead to any meaningful differences in safety, effectiveness, and immunogenicity sought by extrapolation)
- Clinical safety and immunogenicity profiles of the new biosimilar and licensed reference product are compared for indications for use, and the justifications are provided that residual differences will not contribute to safety and effectiveness bias under extrapolation studies
The FDA’s guidance for extrapolation states that data derived from clinical studies should be sufficient to demonstrate purity, potency, safety, and the intended use of the proposed biosimilar product in comparison to licensed biologics.10 The sponsor of biosimilar 351(k) application may apply for licensure for one or more indications for use based on MOAs for which the reference product is licensed. MOAs for safety and efficacy for different indications present a major challenge for extrapolation.25 For instance, MOAs for hormonal protein drugs may be different from antibody drugs. Hormonal protein drugs, such as human growth hormone (hGH) somatropin, generally have similar structure and function as the corresponding endogenous hormones, and their MOAs are considered to be identical with the same binding receptor with identical biological effects. Whereas MOAs for antibody drugs may be different due to complexity of antibody structure, especially the complexity of post-translational modifications, such as glycosylation causing different structural variations of the same antibody whose residual mixture could be different from batch to batch technically making it difficult an exact copy of antibody drug. Structural residual uncertainties in the antibody structure could be detected during the physico-chemical characterization step in the manufacturing process.10 Glycosyation may have potential impacts on the PK/PD of the biosimilar drug antibody.25,26 Remicade (infliximab) biosimilar was approved for inflammatory diseases, such as ankylosing spondylitis (AS), inflammatory bowel diseases (IBD), psoriatic arthritis (PsA), plaque psoriasis (PsO), and rheumatoid arthritis (RA). Clinical studies for the proposed biosimilar Inflectra/Remsima (CT-P13) were conducted for AS and RA and extrapolation to IBD. In these studies, the structural uncertainties reached to lower levels of glycans, which caused to lower antibody-dependent cell-mediated cytotoxicity (ADCC) responses.
Adalimumab biosimilar ABP501, biosimilar of Humira, was approved for rheumatoid arthritis (RA), Juvenile idiopathic arthritis (JIA) in patients approximately 4 years or older, AS, PsA, UC, adult CD, and PsO, while the clinical studies for the proposed biosimilar ABP501 was conducted in PSO and RA. The physico-chemical characterization (with no major residual uncertainties reported) provided justification for extrapolation in comparison to CT-P13.27 The clinical studies supporting the similarity of ABP501 included single-dose PK similarity study in healthy subjects, which was conducted to assess PK parameters simply because these subjects were not under concomitant medications treatments and did not have medical conditions that could potentially affect PK. The study showed PK equivalence assessed by AUCinf and Cmax between ABP501 and US approved licensed product.28 Additional study in subjects with moderate to severely active rheumatoid arthritis and plaque psoriasis demonstrated clinical similarity (safety, efficacy, and immunogenicity) for ABP501and the reference product.28 Additionally, the study results indicated that there was no increased risk to safety, efficacy, and immunogenicity switching from reference product to ABP501.28
Scientific justification for the biosimilar candidate’s extrapolation is based on the totality of evidence presented to demonstrate the analytical characterization of high similarity coupled with high similarity in functional testing for a solid extrapolation justification.9,10 The justification goal for extrapolation of safety is dependent on reducing the residual uncertainty. It is critical that residual uncertainty will not contribute to any significant difference in clinical safety and efficacy in indications sought by extrapolation.
Interchangeability: The FDA requires switching studies (at least three switches) with primary endpoints measuring PK/PD providing assessments for sensitive changes in immunogenicity and efficacy.8 The FDA guidance (FDA 2017b) addresses the use of post-marketing data for a biosimilar product with real-time evidence providing sensitive PK/PD information as a part of post-marketing surveillance process.5,6,8 Current biosimilars include Humira, Enbrel, Rituxan, Remicade, Avastin, Herceptin, and Lantus in their respective lists of top drugs that are widely available through the FDA’s approval process.29 A biosimilar applicant can apply for interchangeability status (1 year interchangeability exclusivity is allowed). To achieve interchangeability approval, the biosimilar applicant is required to show substantial switching studies between the candidate biosimilar and RP. However, the 1-year exclusivity applies only to interchangeability status. Biosimilar candidate product is expected to produce the same clinical result as the RP in any given patient and also not to pose excessive risks to patients if they switch between the RP and interchangeable product without the intervention of the biosimilar product’s prescriber – the interchangeable product may be given in place of the RP at the pharmacy level (Interchangeability Guidance, US FDA 2017a). The FDA expects minimum immunogenicity risk-related outcomes by switching candidate biosimilar and RP products. The PD/PK endpoints become essential sensitivity indicators in the switching studies. The important point of the FDA’s stepwise approach is to consider the outcome supporting biosimilarity assertions in its totality of evidence (ie, filgrastim and bevacizumab interchangeability studies)13,16,22,30
CONCLUSION
Biosimilars have recently emerged as a new class of biologic drug that has the potential to have access to many critical medicines through the reduction of costs. Furthermore, there is need to ensure that new biosimilars are as safe and effective as their innovative counterparts. To date, there are approved biosimilar drugs, spanning a variety of indications for use-from autoimmune disease to growth deficiency, that have fulfilled the needs for the treatment of diseases/abnormalities. It is expected that future biosimilar developments will provide robust pipeline for biosimilars intended to be used in oncology and other severe diseases. At the same time, the manufacturers of biosimilars will have to stay abreast of these biosimilar drugs development and the new technologies, such as the interpretation of data from switching and interchangeability studies. The FDA’s guidance on demonstration of interchangeability emphasizes that alternating use of a proposed biosimilar in comparison to the reference product would not incur more risk than the use of the reference product alone. This article is primarily focused on considerations for the quality system approach to design of studies for clinical applications for designated patient populations and selection of conditions of use.
ACKNOWLEDGEMENT
The views and opinions expressed in this article are those of the author and do not represent official views of the US FDA.
REFERENCES
- Aziz KJ. Drug Monitoring: Modern Approaches to Quality Assurance. CRC Handbook of Analytical Therapeutic Drug Monitoring and Toxicology, 1997;19:335-349.
- Aziz KJ. Global harmonization & quality system application for medical products. Clin Lab Med. 2001;39:76-78.
- Aziz KJ. The FDA’s critical path initiative for medical products. J Clinic Ligand Ass. 2006;29:171-176.
- Aziz KJ. Globalization: Challenges and Solutions (Seventh Annual FDA Inspections Summit). 2012:19-21.
- Aziz KJ. The FDA’s new drug approval process. Drug Development & Delivery. 2016;3:50-56.
- Aziz KJ. The FDA’s quality risk management approach to new drug applications. Drug Development & Delivery. 2019;19:1-4.
- Aziz KJ. The FDA’s design control requirements for biomarkers in drug development. Drug Development & Delivery. 2019;3:39-43.
- Aziz KJ. Biosimilar drug development and approval process. J Pharmaceut Sci & Emerg Drugs. 2019;7:2;1-5.
- Cohen H. Biosimilar development: approval of biosimilar medicines through totality of evidence. Drug Development & Delivery. 2019;19:40-45.
- Aziz KJ. Biosimilar biological products: development & applications. Drug Development & Delivery. 2019;19:1-6.
- Rader RA. An analysis of the US biosimilars development pipeline and likely market evolution. Bioprocess Inter. 2013;11:16-23.
- Putnam WS, et al. Pharmacokinetic, pharmacodynamic and immunogenicity comparability assessment strategies for monoclonal antibodies. Trends Biotechnol. 2010;28:509-516.
- Desai K, et al. Confirmation of biosimilarity in a pharmacokinetic/pharmacodynamic study in healthy volunteers for an analytical highly similar pegfilgrastim. Clin Pharmacol Drug Dev. 2016;5:354-363.
- Ducourau E, et al. Antibodies toward Infliximab are associated with low Infliximab concentration at treatment initiation and poor Infliximab maintenance in rheumatic diseases. Arthritis Res Ther. 2011;13:R105.
- Hobbs KF, et al. Rheumatoid arthritis disease measurement: a new old idea. Rheumatol. 2012;51:21-27.
- Moots R, et al. Switching between reference biologics and biosimilars for the treatment of rheumatology, gastroenterology, and dermatology inflammatory conditions: considerations for the clinician. Curr Rheumatol Rep. 2017;19:37.
- NIH National Cancer Institute. Using the Immune System in the fight against Cancer: Discovery of Retuximab. 2014, available at : https://www.Cancer.gov/research/ progress/discovery/blood cancer. Accessed 22 Nov, 2017.
- Casadevall N, et al. Pure red-cell aplasia and antierythropoietin antibodies in patients treated with recombinant erythropoietin. NEJM. 2002;346:469-475.
- Steenholdt C, et al. Severe infusion reactions to infliximab: etiology, immunogenicity and risk factors in patients with inflammatory bowel disease. Aliment Pharmacol Ther. 2011;34:51-58.
- Kalden JR. Immunogenicity and loss of response to TNF inhibitors: implications for rheumatoid arthritis treatment. Nat Rev Rheumatoid. 2017;13:707-718.
- Maas C, et al. A role for protein misfolding in immunogenicity of biopharmaceuticals. J Biol Chem. 2007;282:2229-2236.
- Jahn EM. How to systematically evaluate immunogenicity of therapeutic protein. New Biotechnol. 2009;25:280-286.
- Perdriger A. Infliximab in the treatment of rheumatoid arthritis. Biologics. 2009;3:183-191.
- Brinks V. Immunogenicity of biosimilar monoclonal antibodies. GaBI J. 2013;2:188-193.
- Suzuki MK, et al. Therapeutic antibodies: their mechanism of action and the pathological findings they induce in toxicity studies. J Toxicol Pathol. 2015;28(3):133-139.
- Liu L. Antibody glycosylation and its impact on the pharmacokinetics and pharmacodynamics of monoclonal antibodies and Fc-fusion proteins. J Pharm Sci, 2015;104(6):1866-1884.
- FDA Guidance for Industry: scientific considerations for CT-P13 Infliximab biosimilar. 2016(a). https:www.fda.gov/ downloads/drugs/guidances/ucm 484859. Pdf.
- FDA briefing for Adalimumab. (https//www.fda.gov/downloads/drugs/guidances/ucm 397017.pdf. Accessed Jan 18, 2017.
- US Food and Drug Administration (FDA) Biosimilar Product Information (2019). Available at https://fda.gov./drugs/biosimilars/biosimilar-product-information. Accessed May 7, 2019.
- Ha K, et al. Statistical considerations in evaluating a biosimilar product in an oncology clinical study. Clin Cancer Res. 2016;22:5167-5170.
To view this issue and all back issues online, please visit www.drug-dev.com.
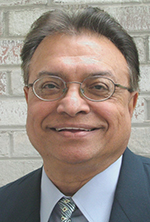
Dr. Kaiser J Aziz completed a 30-year career with the FDA as a clinical regulatory scientist and manager of drug and device evaluations, approvals, reengineering, standards, good manufacturing and quality system applications. He worked with individual and industry organizations in design and total product life cycle (TPLC) approaches to premarket applications for medical devices, pharmaceuticals, and combination products (510ks, NDAs, and PMAs). Prior to joining the FDA, he developed and implemented quality assurance standard operating procedures and protocols for medical diagnostic systems in hospitals, physicians’ offices, and clinical reference laboratories. During his tenure at FDA, he served as an adjunct faculty in the Department of Medicine and Physiology, NIH Graduate School, where he developed and taught courses in Clinical Pharmacology, Toxicology, Therapeutic Drug Monitoring, and Clinical Laboratory Medicine. Currently, he serves as an adjunct faculty at Virginia Tech’s Medical HACCP Alliance Program, where he teaches Quality Risk Management courses and workshops using HACCP principles for the pharmaceutical industry. He was an invited guest editor on Nanotechnology and Clinical Trials in the journal of Clinical Ligand Assay. He is the author of book chapters, textbooks, and over 70 publications in professional and trade journals. His expertise includes Quality System Approach to Medical Device and Pharmaceutical Premarket Applications.
Total Page Views: 3454