Issue:October 2023
BIOLOGICS DEVELOPMENT - Five Steps to a Robust Cell Line Development Process
INTRODUCTION
Biologic production is accelerating, with an increasing number of these essential treatments reaching the market as therapies for a myriad of diseases, including Crohn’s disease, hemophilia, diabetes, and cancer.1 This increasing demand is reflected in the biologics market, which is predicted to expand at a compound annual growth rate of 8.1% to reach $535.5 billion by 2028.2 As the demand for biologics rises, ensuring robust cell line development is essential.
Working to construct a robust cell line that will lead to efficient, reliable, and reproducible production is the foundation of biologic manufacturing. Cell lines often have distinct associated characteristics that may be beneficial for producing one product but detrimental to another. When deciding between cell lines, each must be carefully considered for the desired biologic to ensure a suitable choice.
Cell line development is the use of various techniques to optimize a cell line for the production of a stable target biotherapeutic in high yield. Not taking the time to implement a robust cell line development process can lead to difficulties down the manufacturing pipeline, which wastes time and increases costs, and could even halt the timeline, as the process may need to be repeated and optimized until the challenges are overcome.
But what steps can be taken to help ease these challenges? The following discusses the critical steps for designing and implementing a robust cell line development process to help overcome the obstacles that often hinder biologic production. By taking the time to understand the cell line, the biologic, and by using advanced synthetic biology techniques to tailor the method to the desired biologic, a proactive approach for robust CLD can be devised.
THE FOUNDATION OF CELL LINE ENGINEERING
Cell line engineering can be challenging, and issues can arise throughout manufacturing. Examples include cell line instability, low yield, low product purity, problems with reproducibility and scalability, or regulatory compliance issues. Fortunately, a number of methods can be adopted to overcome these issues, such as testing various cell lines, generating stable pools, completing extensive and high-throughput screening for clone selection, and using synthetic biology to introduce beneficial characteristics into a cell line. These five techniques, used to generate a robust cell line, are explored in depth further.
1: Choosing the Appropriate Cell Line
Deciding upon the cell line at the earliest possible point is key, as it will impact all subsequent development and manufacturing steps. The conditions for cell growth, expression, and product isolation depend on the selected cell line. As well as impacting factors, such as product quality, quantity, reproducibility, and efficiency, a robust cell line development platform must also offer scalability. Therefore, it forms the foundation of biologic development and manufacturing.
Many cell line options have been successfully used for biologic development projects. Chinese hamster ovary (CHO) cells are a logical choice, with more than 70% of recombinant proteins produced using this line.3 With a clear history that sets the basis of documented traceability and ICH compliance, CHO-K-derived cell lines are a safe and easy-to-use option with a track record for gaining regulatory approval. A number of advantages make CHO lines a common choice, including the following:3
- Protein folding and post-translational modification processing, such as glycosylation, are closely conserved in nature with human proteins and so are more likely to be accepted by the human system.
- The lines are uniquely tolerant to changes in pH, oxygen levels, pressure, or temperature throughout manufacture, so they can be tailored to the protein at hand.
- The lines are able to grow in serum-free suspension cultures with high cell concentrations, which eases scale-up as it is preferred for large-scale bioreactors.
- There is previous understanding of CHO cell lines with extensive literature available and a regulatory track record, which can help accelerate timelines for approval.
As listed, CHO cells offer several advantages, making them an apt choice. The CHO cell line, however, does have limitations, such as restricted growth and low productivity (when expressing complex artificial molecules), but through genetic engineering, it can be tailored for the desired application.4
2: Productivity Improvement Techniques for Genetic Engineering & Non-genetic Optimization of the Cell Line
Biologics are complicated molecules, and past research has shown the CHO cell line may not have the capabilities to produce a particular compound in high yields and at high quality without first being engineered to enhance the expression of the gene of interest to overcome low productivity issue.5
Alterations to the core DNA must be completed to impart specific characteristics to the cell line. This is done using genome editing techniques, such as CRISPR Cas, Zinc Finger Nucleases (ZFNs), or Transcription Activator-Like Effector Nucleases (TALENs), to implement specific characteristics.6 Genetic engineering of the CHO line has been shown to fundamentally alter the function of the molecules expressed.
Biologic expression becomes inherently more difficult as complexity grows. The biopharmaceutical industry is moving toward more intricate molecules, such as next-generation antibodies and protein-based drugs like fusion and multi-specific proteins. However, these revolutionary therapeutics often have lower expression levels in CHO cells as they are artificially designed. While effective for their designed purpose, how these proteins are devised can lead to issues with decreasing solubility and increased aggregation. Engineering can be used to address these issues by introducing the genes for chaperone production (additional proteins that are produced during expression and aid protein folding) into the cell line to help with protein solubility and, in turn, increase product yield. Alternatively, engineering cells to improve the volume of recombinant protein production at low temperatures can also benefit CHO-based expression systems.7
Non-genetic cell culture optimization of an existing product-expressing CHO cell line can also improve productivity, for example, by using a low temperature, pH control, media selection, or a feeding strategy. These cell culture process optimizations are synergic to maximize the expression potential of native CHO production cell lines. Different clones have different responses toward cell culture process changes, and therefore, design of experiment (DoE) is necessary to evaluate multiple CHO cell lines. Developing a scale-down cell culture model representative of at-scale production forms the basis of the DoE.
3: Reducing Variability Between Product Batches
Batch variability leads to unreliable biologic production. Changes in product yield and quality for each batch can result in missed targets and hard-to-predict scales. Variability can be the result of numerous circumstances, from varying growth conditions or materials to cell line composition. Therefore, working to construct a robust cell line and adhering to strict conditions is essential.
Cell line engineering is only made possible by using techniques for efficient transfection and cell enrichment. These high-throughput methods test the viability of an engineered cell line with the product vector to see if the cell engineering has implemented the desired beneficial characteristic (such as increased product yield or solubility) for biologic production.
There are a number of different approaches for inserting the product vector into the target cells, including those based on viral, chemical, and physical transfection for integration. Choosing the best transfection technique for both the vector and cell line is essential to ensure robust vector uptake and limit cell variability.7 Cell enrichment is a method of single cell sorting to isolate positive cells, such as using antibody-based cocktails or flow cytometry.8 Innovative technologies, such as single-cell printers and high-resolution imagers, make this high-throughput screening possible to ensure monoclonality and increase clone screening efficiency. The method used depends on the application, but for biologic production, a method that leads to high purity is essential.
Optimizing transfection techniques is just one method for limiting variability. An optimized cell line development workflow is also essential; furthermore, a robust single-cell cloning technology minimizes the manufacturing batch-to-batch variability based on the selected clone. Finally, ensuring cell culture medium, growth conditions, extraction techniques, and other production methodologies are meticulously replicated is crucial to reducing variability and ensuring reproducibility.
4: Biologic Analytics Aid Production Viability
Biologic analytics are a powerful tool that can significantly aid the development of stable cell lines. Using intensive biologic analytics early in the cell line development process is essential to fully characterizing and understanding the nature of the biologic. With these characteristics in mind, biologics developers can design production methods knowing the protein will not be harmed by the techniques used during manufacture and that the method is designed to impart stability to the molecule. This circumvents the need for extensive and complex formulations post-production to repair the protein.
Aided by high coordination and free-flowing communication between the analytical and biotechnology groups, this communicative relationship helps to ensure meticulous characterization and top-level visibility, which is especially important for emerging types of molecules (various types of fusion and multi-specific proteins), where less information is known.
5: Scale-up for Market
After extensive optimization and analysis for the reliable production of a biologic, the process then needs to be scaled up to produce enough product for the patient population. Scaling a process up can have unforeseen effects on a biologic and can significantly impact the yield and quality of the product. However, this can be eased by adopting specific intermediary techniques to help aid visibility. For example, using scale-down models to evaluate clones in 50-mL bioreactor spin tubes mimics the larger-scale process so it can be used to predict how a clone will perform within a large-scale bioreactor. Any issues or changes identified when using the 50-mL bioreactor can then be addressed before scaling up to the commercial scale.
As previously discussed, implementing a robust cell line development process helps to reduce batch variability, increase product yield and purity, offer scalability, and reduce production costs. Future risks are also mitigated, and the timeline is less likely to suffer from development and manufacturing delays. However, this can still be very challenging, especially when a highly complex biologic is involved.
A SUPPORTIVE PARTNER AIDS ROBUSTNESS
Working alongside a supportive partner can help ensure a cell line strategy is effective and can aid in maintaining tight timelines. Experienced contract development and manufacturing organizations (CDMOs) have the expertise to help overcome the challenges often encountered at this early stage. They can help with implementing techniques to enhance the stability and productivity of protein drugs for a desired use.
LOOKING TO THE FUTURE
2022 saw a milestone in biologics development: the number of biologic drugs brought to market matched those of new molecular entities (NMEs) for the first time. This growth will only continue, and it is likely biologics will outpace NMEs in the near future.9 A growing understanding of synthetic biology, enhancing our ability to perform complex genetic engineering, facilitates access to increasingly complex and hard-to-work-with biologics to tackle complex diseases.
Therefore, working to implement robust, scalable, and reproducible cell lines at speed is the foundation of biologic production and is essential to keep up with the accelerating biologic market. Investing in new technologies and techniques to streamline CLD will make success more certain and meet customer needs.
REFERENCES
- Biologics: Types, Treatable Conditions, Mechanisms, Side Effects (verywellhealth.com).
- Biologics Market Report, Size, Growth and Forecast 2023-2028 (imarcgroup.com).
- Dumont, J., Euwart, D., Mei, B., Estes, S., & Kshirsagar, R. Human cell lines for biopharmaceutical manufacturing: history, status, and future perspectives, Critical Reviews in Biotechnology, 36:6, 1110-1122, (2016). https://doi.org/10.3109/07388551.2015.1084266.
- Fischer, S., Handrick, R., & Otte, K. The art of CHO cell engineering: A comprehensive retrospect and future perspectives. Biotechnology Advances, 33:8, 1878–1896, (2015). https://doi:10.1016/j.biotechadv.2015.10.015.
- Noh, S. M., Sathyamurthy, M. & Lee, G. M. Development of recombinant Chinese hamster ovary cell lines for therapeutic protein production. Current Opinion in Chemical Engineering 2, 391–397 (2013). https://doi.org/10.1016/j.coche.2013.08.002.
- Dangi, A. K., Sinha, R., Dwivedi, S., Gupta, S. K. & Shukla, P. Cell line techniques and gene editing tools for antibody production: A Review. Frontiers in Pharmacology 9, (2018). https://doi.org/10.3389/fphar.2018.00630.
- Chong, Z. X., Yeap, S. K. & Ho, W. Y. Transfection types, methods and strategies: A technical review. PeerJ 9, (2021). https://doi.org/10.7717/peerj.11165.
- Cell Enrichment | SpringerLink.
- Senior, M. Fresh from the biotech pipeline: fewer approvals, but biologics gain share. Nat Biotechnol 41, 174–182 (2023). https://doi.org/10.1038/s41587-022-01630-6.
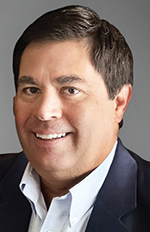
Robert Gustines is Senior Vice President Commercial Operations at Bora Pharmaceuticals. He has more than 18 years of experience in business development and marketing in the biopharma/pharmaceutical industry. During this time, he has consistently driven revenue and commercial capabilities and enhanced the performance of BD teams in high-growth, proactive, and technology-based environments. He has held several senior roles in Business Development.
Total Page Views: 3254