Issue:May 2013
AUTOLOGOUS CELL THERAPIES - Development of Autologous Cell Therapies: Keys to Clinical & Commercial Success
INTRODUCTION
Throughout the past decade, there has been a steady increase in studies of cell therapies for clinical use, with approximately 47 industry-sponsored clinical trials in pivotal or late stages using autologous cells.1 Autologous cell therapies involve taking a cell sample from a patient and manipulating those cells, and then administering them back into the patient. Such therapies have been shown to aid in the repair of tissues in many therapeutic areas, including cardiovascular disease, peripheral arterial disease, liver disease, diabetes, neurodegenerative disorders, bone repair, and spinal cord injuries.2-5
The patient-specific nature of autologous cell therapies can make large-scale production extremely challenging because a separate batch must be produced for each patient. Companies often struggle to scale up manufacturing as necessary in clinically and commercially viable ways. Cell therapies also often carry higher manufacturing risks than most small molecule pharmaceuticals. An error in the production of an autologous cell therapy directly translates into a failed treatment for a patient. And the often complex cell manufacturing process must meet both FDA regulations and the requirements of a successful business model.
Some companies are exploring the use of allogeneic cell therapies, which derive treatments or doses for multiple patients from the cells of a single donor. This approach can help to make manufacturing more efficient, leading to potential cost savings. However, the use of cell therapies derived from one donor in a different recipient must address the risk of immune response and rejection. With autologous therapies, patients receive cells that come from their own body, which virtually eliminates the risk of rejection. In addition, cells for allogeneic therapies must be extensively cultured and cryopreserved for long-term storage. This prolonged culture increases the risk of cell transformation (eg, tumorigenicity) and often the frozen product requires further manipulation prior to administration.
Many autologous cell therapies are produced with ex vivo culturing to increase the number and/or modify the therapeutic cells within the patient’s cell sample. In this way, therapies derived from a cell sample will include a higher number, ratio, or type of cells known or believed to play a role in disease treatment. This process can add additional complexity to manufacturing because cell culture conditions must be optimized and extensively tested and monitored to maintain the regenerative potential of the cell.
Drug developers targeting autologous cell therapies must identify the optimal strategies to commercialize cell therapies safely while being able to manufacture therapies at a high volume without compromising quality. Aastrom Biosciences, one of the approximately 47 companies with an autologous cell therapy in late-stage development, has developed the innovative processes necessary to reduce risk throughout the manufacturing process. The company is now preparing for future commercialization for its investigational therapy ixmyelocel-T, derived from a patient’s own bone marrow stem cells. The company has advanced ixmyelocel-T into Phase III clinical development in critical limb ischemia (CLI), which is a severe form of peripheral arterial disease.
OVERCOMING CHALLENGES IN CELL COLLECTION
Bone marrow contains two important types of stem cells: hematopoietic stem cells (HSCs) and stromal cells, which include mesenchymal stem cells (MSCs), multipotent stromal cells, and endothelial progenitors. Bone marrow stem cells are known to support cell regeneration and regrowth, and are being tested in several cell therapies, including ixmyelocel-T. Yet only 1 in 10,000 to 20,000 cells in bone marrow are HSCs, while MSCs are ten-fold less abundant.6,7 Taking a large-volume sample of bone marrow is one approach used to ensure that a sufficient supply of these essential but rare stem cells is present in the cell sample. However, large-volume collection is typically painful for patients, requires hospitalization, and can cause side effects that include bone marrow inflammation.
Aastrom has identified a way to take a smaller bone marrow sample (about 60 mL) from the patient and then expand the hematopoietic and stromal cells during the manufacturing process using ex vivo culturing. The bone marrow sample is withdrawn from the patient under local anesthesia during a 20-minute outpatient procedure.
Though a smaller sample is beneficial to patients, the quality of the cell sample could be compromised if the sample is not collected properly. To achieve controlled outcomes and the best possible cell sample for manufacturing, Aastrom has developed a unique collection process, a detailed training program, and a kit with all of the materials for collection and shipping of the bone marrow to the manufacturing site.
STEPS TO REDUCE MANUFACTURING RISK
Once a cell sample is collected, it is typically shipped at a controlled shipping container to a centralized manufacturing site for processing. There are many manufacturing risks companies need to address once the sample arrives on site, including potential cross-contamination, cell damage, or improper labeling and record keeping that could lead to loss of patient identity. Because cellular products are regulated by the FDA as biologics, they require a Biologics License Approval (BLA) after commercialization. They also need to comply with Good Manufacturing Practice (GMP) regulations.
To minimize risk, there are several steps that can be taken in alignment with GMP regulations. Using closed-system technology with single-use disposables is one way to help minimize contamination risks. At Aastrom, each cell sample is placed in a single-use, sterile disposable cell cassette, and then an instrument uniformly distributes the cells over the culture surface for optimal growth. Each cell cassette also has an electronic memory device that identifies the cell product, instructs instruments through the cell production process, records the cassette status, and collects data. All instruments continually update status so that when a cassette is inserted into the incubator unit or when power is restored after interruption, the device knows exactly which point the cassette has reached in the production process. The electronic device helps to reduce the risk of mix-ups and operator errors.
Each cell cassette at Aastrom is placed into a dedicated incubator during the 12- day incubation process. As a therapy is prepared, the system controls all aspects of the culturing process, including temperature, culture medium exchange, and gas exchange and confirms that all functions are operating correctly. It can also automatically place a process into a “safe state†in the rare event of an equipment or utility failure.
When the incubation is complete, Aastrom manufacturing personnel then harvest the cells from the cassette, again using an automated process, which helps to achieve the highest attainable levels of reliability, consistency, and cost-effective manufacturing.
POST-CULTURE PROCESSING
Typically, cells harvested from a cell culture are not suitable for direct patient administration. The cells need to first undergo several manufacturing steps to remove process residuals, achieve an appropriate product volume, and formulate the cells for delivery, which could include excipients to extend shelf-life. These steps are not easy to integrate into product manufacturing. The post-culture phase is also relatively time-consuming and subject to increased risk of error, contamination, and excessive holding times. In the case of a complex mixture of cell types, as in ixmyelocel-T, this process can cause a shift in the cell profile. And the transfers in this phase can result in cell loss or decreased cell viability.
Traditional approaches to cell harvesting produce cells mixed with culturing byproducts that need to be washed away in an additional processing step before cells can be sent back to the patient. Aastrom employs its automation system to drain away the culture medium and rinse the cells within the bioreactor before the cells are harvested. This removes two cell transfer steps and also leads to a lower rate of cell loss and cell damage. The company’s closed system methodology also helps to reduce product volume and introduce storage excipients to prepare the therapy in a form suitable for administration. The system is not highly complex and also helps make the manufacturing process more efficient.
FINAL PRODUCT TESTING
Testing a cell therapy before it is injected back into a patient can be challenging for several reasons. First, all tests must be conducted rapidly because ixmyelocel-T has a 72-hour shelf-life. They should also be relatively inexpensive because the same tests will be repeated for every dose. The tests should only require a limited amount of cell sample volume to avoid excessive product loss. And testing must be able to screen a complex product composition.
In final product testing, critical quality attributes of the final product must be confirmed, including identity, potency, viability, dose, sterility, and purity. Careful selection of test parameters, testing method, and sample requirements for each of these attributes is essential to minimize product loss, test time, and cost.
COST SAVINGS & FUTURE EFFICIENCY CHALLENGES
While most pharmaceutical manufacturing processes, as well as allogeneic cell therapies, can take advantage of the efficiencies of “sharing†a single production batch among multiple patients, this is not the case for autologous cell therapies. The core steps for manufacturing cannot be shared, so companies need to look into other ways to achieve efficiencies. For example, laborsaving strategies, computerization, process automation, and engineered integration can each drive cost-savings. Increasing the number of cell products that can be manufactured in a given amount of time is necessary and often referred to as “scale out†rather than scale up.
Simplifying and integrating manufacturing steps also provides significant cost-savings. Aastrom’s integration of washing and cell harvest is one example. The integration of automated cell sampling or quality control into manufacturing can reduce labor and processing times as well. This would additionally help to improve consistency. High-volume screening and multiplexing technologies in pharmaceutical companies might also be examined to help to address challenges in high-volume autologous cell therapy manufacturing.
REFERENCES
1. Buckler L. (2011, Dec. 9). Late-stage industry sponsored cell therapy trials. Retrieved from: http://celltherapyblog.blogspot.ca/2011/12/active-phase-iii-or-iiiii-cel-therapy.html.
2. Orlic D, Kajstura J, Chimenti S, Bodine DM, Leri A, Anversa P. Bone marrow stem cells regenerate infarcted myocardium. Pediat Transplant. 2003;7(Suppl. 3):86-88.
3. Strauer BE, Brehm M, Zeus T, Kostering M, Hernandez A, Sorg RV, Kogler G, Wernet P. Repair of infarcted myocardium by autologous intracoronary mononuclear bone marrow cell transplantation in humans. Circulation. 2002;106 (15):1913-1918.
4. Tateishi-Yuyama E, Matsubara H, Murohara T, Ikeda U, Shintani S, Masaki H, Amano K, et al. Therapeutic angiogenesis for patients with limb ischaemia by autologous transplantation of bone-marrow cells: a pilot study and a randomized controlled trial. Lancet. 2002;360:427-435.
5. Taguchi A, Ohtani M, Soma T, Watanabe M, Kinosita N. Therapeutic angiogenesis by autologous bone-marrow transplantation in a general hospital setting. Eur J Vasc Endovasc Surg. 2003:25(3):276-278.
6. Weissman LL. Stem cells: units of development, units of regeneration, and units in evolution. Cell. 2000;100:157-168.
7. Shi Y, Hu G, et al. Mesenchymal stem cells: a new strategy for immunosuppression and tissue repair. Cell Res. 2010:20:510-518.
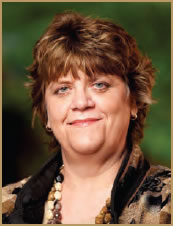
Dr. Ronnda L. Bartel serves as Chief Scientific Officer at Aastrom Biosciences and is responsible for the scientific direction of the company, including research, development, and technical operations. She has more than 20 years of research and product development experience and most recently was Executive Director, Biological Research at MicroIslet and Vice President, Scientific Development at StemCells Inc. Earlier in her career, she was Senior Principal Scientist and Director of Research at Advanced Tissue Sciences and was involved in the development and approval of some of the first cellbased products approved by the FDA. She has also worked as Senior Director, Science and Technology at SRS Capital, LLC evaluating life science investments and has held positions in clinical development, drug delivery, business development, and manufacturing. Dr. Bartel earned her PhD in Biochemistry from the University of Kansas, has completed post-doctoral work at the University of Michigan, and earned her BA in Chemistry and Biology from Tabor College.
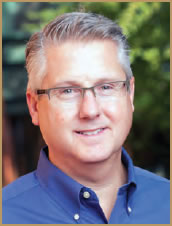
Tod Borton has served as the Vice President of Technical Operations at Aastrom Biosciences since 2006 and has more than 25 years of product development and quality experience in the domestic, international, biotechnology, and medical device industries. Prior to joining Aastrom, he held the position of Director of Quality at Terumo Cardiovascular, where he was responsible for quality assurance, document control, and sustaining engineering. Prior to Terumo he held the position of Vice President, New Product Development and Advanced Engineering at Pall Corporation, where he developed and commercialized many biotech and medical filtration devices. Mr. Borton has authored many patents in the area of biotechnology and medical filtration device technologies and earned his BS in Plastic Technology from Eastern Michigan University.
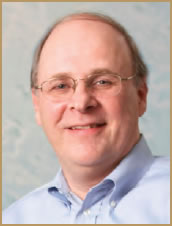
Brian Hampson has over 25 years of experience in the development and engineering of novel culture systems, cell processes, and related control system and automation technology. He has held several executive and technical management positions at Aastrom Biosciences and was a Principal Leader in the development and engineering of the AastromReplicell Cell Production System, a first-of-its-kind automated manufacturing platform for culture-derived cell therapy products. He currently holds the position of Senior Engineering Fellow, where he is responsible for strategic planning and development of high-throughput technology for cost-effective, commercial-scale manufacture of Aastrom’s cell therapy products. Previously, Mr. Hampson was at Charles River Laboratories, where he managed a number of programs to develop and commercialize novel bioreactor systems to support large-scale biomolecule production. Mr. Hampson has authored or co-authored a variety of abstracts, publications, invited presentations, grants, and patents. He earned Bachelors and Masters degrees in Engineering from Cornell University.
Total Page Views: 7062