Issue:November/December 2021
APTAMER TECHNOLOGY - From Postal Codes to GPS: Building Better Drug Conjugates With Aptamers
INTRODUCTION
Orthoclone OKT3 was the first therapeutic monoclonal antibody brought to market in 1986 for the treatment of kidney transplant rejection.1 Following this, the biotherapeutic market has continued to expand, representing the fastest-growing pharmaceutical market sector. Predicted annual growth of 14% with annual sales of over $120 billion in this sector compares favorably to growth of just 6.8% for traditional pharmaceuticals.2,3 Indeed, last year, five of the top-ten best-selling drugs were antibody therapeutics, and antibody technology has a proven track record in developing effective therapies with good safety profiles across oncology, hematology, cardiology, immunology, and autoimmunity.4-6 The increased interest in this sector has spurred advances in immunotherapy, gene and cell therapies, and affinity reagent technologies. However, these advances have also revealed limitations in antibody technology that prevent these molecules from meeting the developing potential of precision medicine.2,3 Such limitations include the large size of antibody molecules (Figure 1), which hampers their ability to penetrate tissues in vivo, and their structural complexity, which requires expensive and difficult to optimize mammalian manufacturing systems.
A new breed of affinity binders that can be used as an alternative to antibody therapeutics has the potential to reshape the industry, delivering new medicines with improved safety and efficacy profiles and reduced healthcare costs.
ANTIBODY-DRUG CONJUGATES: THE PROBLEMS
Conjugating drug molecules to an affinity ligand produces targeted therapies that can enhance the selectivity and hence the performance of the therapeutic agent while simultaneously reducing off-target interactions, which lead to adverse side effects.7 Antibody-drug conjugates (ADCs) allow the combination of the targeted precision of antibodies with:
- the potency of small molecules for targeted therapy
- oligonucleotide therapeutics (such as siRNA) – for the targeted cellular delivery of gene therapy
- an additional affinity reagent as a bispecific modality for increased therapeutic targeting and receptor activation
From the introduction of the first ADC in 2001, these molecules have undergone multiple rounds of refinement, including developing new toxic payloads, increasing the linker stability, and assessing the potential for antibody alternatives.8 Though the high target binding affinities of monoclonal antibodies reduce off-target effects, the large size of these biologics has been shown to restrict their diffusion through tissue in vivo and limit the accessibility of ADCs to certain epitopes.9 Their high molecular weight also reduces the rate of renal clearance. While this can be beneficial in that it reduces dosing frequency, it can also be detrimental in that long circulation times increase the potential for off-target effects and the development of anti-drug antibodies.
Antibodies are composed of multiple polypeptide chains that are variably glycosylated and connected through numerous disulfide bonds. This structural complexity poses a significant challenge to the scale-up of their manufacture, requiring expensive mammalian expression systems and often leading to batch consistency issues.10 Additionally, for ADC production, the final antibody must be further modified with the therapeutic payload. Site-specific chemistries, such as maleimide linkages on cysteine residues, can still lead to problems in generating a homogenous product due to the number of cysteine residues present in the antibody molecule and their varying accessibility and chemical reactivity. While there has been considerable progress in this area, significant challenges remain in controlling the antibody:drug ratio and purification of the final product.
Delivery of the final ADC cargo to the cell’s cytoplasm for its effector function is not straightforward with antibodies. Due to the large size of antibodies, cellular internalization can be retarded, requiring highly stable linkers to prevent premature linker cleavage and release of the cargo before it reaches the intended site of action.11
ADVANCING BIOLOGICS WITH APTAMER TECHNOLOGY
The use of nucleic acid aptamers as the targeting moiety overcomes many of these pitfalls. Aptamers are small, single-stranded oligonucleotide molecules isolated from large libraries, using highly tunable in vitro processes. Based upon their nucleotide sequences, aptamers form a variety of structures and can be selected to specifically bind to their target molecules in the same way as antibodies. However, aptamers also offer manufacturing advantages as they are generated via well-defined solid-phase synthetic processes, for batch consistency and simple modification with a range of cargo.12 Due to their small size, aptamers have a short half-life in vivo, offering reduced toxicity concerns compared to antibodies, which can stay in the patient’s circulation for weeks. To delay renal clearance, strategies must be adopted, such as PEGylation, which increase half-life where required.13 Aptamer-drug conjugates (ApDCs) are offering new potential for this therapeutic approach to provide effective treatments for a range of diseases.
The first aptamer therapeutic, pegaptanib sodium (Macugen) was licensed in 2004 for the treatment of neovascular (wet) age-related macular degeneration.14 A further 10 aptamer therapeutics have successfully entered clinical development for conditions ranging from oncology and inflammation to diabetes and coagulation.
NOXXON Pharma’s unique aptamer-based Spiegelmers® are L-enantiomers and consequently not subject to nuclease activity for increased retention. Ongoing trials for Spiegelmer therapeutics include Phase 1/2 trials for the treatment of pancreatic cancer and brain cancer in different combinations and Phase 1 trials for the treatment of solid tumors.15 Iveric Bio have progressed their complement 5 targeting aptamer-based therapeutic for the treatment of age-related macular degeneration to Phase 3, and for the treatment of autosomal recessive Stargardt disease to Phase 2.16
The advantages that aptamer therapeutics hold over standard antibodies and antibody-related binders, have led to them being investigated as direct therapeutics and as delivery vehicles for a range of cargo.17 Many examples of ApDCs have been generated with cargo ranging from chemotherapeutics and photosensitizers to siRNA (Table 1).7
BREAKING THE BARRIERS TO CLINIC
Despite more than three decades since the discovery of the first aptamers, development of these molecules through the clinic has been limited. This lag period is not unexpected in the uptake of a new technology, and many of the perceived limitations of therapeutic aptamers have been addressed through new advances:
Susceptibility to ubiquitous nucleases within the body – The issue of stability has been largely overcome by modifying the nucleic acid backbone with the substitution of 2’-hydroxyl groups in RNA aptamers with 2’-fluoro, 2’-amino or 2’-O-methyl groups, and through 3’-end capping with inverted thymidine, other blocking molecules or the circularisation of aptamers.30
Rapid clearance by renal filtration – Chemical conjugation with high molecular weight polyethylene glycol (PEG), cholesterol or other carrier molecules helps to reduce the rate of renal clearance and improve aptamer retention in vivo. In some cases this strategy has been shown to extend aptamer half-life beyond 48 hours, thus improving their pharmacokinetic properties.30 However, for situations such as the development of imaging reagents or “hit-and-run” treatments with highly toxic payloads (where long retention times are detrimental) rapid clearance may be beneficial for ApDCs. The ability to tune aptamer therapeutic half-life in this way is beneficial.
DEVELOPING APTAMERS FOR DRUG DELIVERY
As aptamer technology is increasingly explored for its therapeutic potential, there are a number of additional advantages to these molecules that make them particularly efficient for drug delivery.
Biomarker-Free Selection & Screening
The in vitro selection process for cell-targeting aptamers allows the discrimination of cell phenotypes, such as diseased versus healthy. Each cell type can be included in the aptamer selection strategy as part of multiple positive and negative selection rounds, removing the need for a priori knowledge of disease-specific biomarkers. Aptamers that bind to the diseased cells and not to the healthy cell population can subsequently be used to pull-down the specific biomarker that they target.31 In this way, aptamer selection can be used for biomarker discovery and validation in one complete step.
Simple, Cost-Effective Manufacturing
Aptamers can be synthesized using well-defined and characterized chemical processes, with no requirement for cell systems. This makes aptamer production more cost-effective and simpler to scale up than comparative protein-based therapeutics. For ApDCs that are delivering oligonucleotide therapeutics, such as siRNA or antisense oligonucleotides, there is also the potential to synthesize the ApDC as a single contiguous molecule, removing the need for additional conjugation and purification of the final aptamer with the drug cargo. This greatly reduces the production costs, increases standardization of the product and improves yields relative to processes requiring post synthesis conjugation.
Rapid Internalization for Drug Delivery
As aptamers are approximately one-tenth the size of antibodies, they can be readily taken up by cells through a number of mechanisms, most commonly endocytosis and micropinocytosis.32 Aptamer uptake is ultimately determined by the specific interaction with their target, making them very appealing for the delivery of cargo to the cell interior for targeted chemotherapy or gene therapy applications.
Delivery to Challenging Tissues In Vivo
Delivery of therapeutics across the blood-brain barrier has long been challenging, resulting in limited treatment options for many brain and central nervous system diseases. Many targeted antibody therapeutics for neurological conditions have failed to show clinical benefit and pose serious risk of eliciting an immune response. Aptamers have shown to be a promising class of therapeutic for the potential treatment of brain disorders, as they are small, non-immunogenic, and able to permeate the blood-brain barrier with relative ease compared to larger antibody molecules.28,33
A second challenging site for targeted delivery of oligonucleotide therapeutics is the kidney.34 Despite increased approval of oligonucleotide therapeutics, targeting these molecules to extrahepatic tissues remains challenging. Due to the small size of oligonucleotides, targeting to the kidney is difficult as it promotes the rapid renal filtration of the intended therapeutic, limiting the potential effect. To overcome this problem, siRNA and antisense oligonucleotides can be conjugated to aptamers that are both highly specific for targeted delivery, and with high affinity to prevent the therapeutic being cleared from the kidney. Aptamer Group is currently working in collaboration with AstraZeneca to develop such solutions for the treatment of kidney disease using aptamer technology.35
LOOKING TO THE FUTURE
As the potential of biotherapeutics continues to expand and the limitations of antibody technologies are increasingly being highlighted, a new breed of oligonucleotide-based affinity binders is showing their value as more molecules progress steadily toward clinical application. Aptamers offer many advantages over antibodies and protein-based binders, including ease of formatting and production, improved cell internalization, and the ability to target new diseases, such as in the CNS. With many of the perceived limitations of aptamers having been successfully addressed, aptamers are coming of age. These novel therapeutics have the potential to deliver effective treatments that can transform patients’ lives whilst offering essential cost savings for healthcare providers who urgently need alternatives in the face of the high cost of antibody therapeutics.
REFERENCES
- Hooks MA, Wade CS, Millikan WJ Jr. (1991) Muronomab CD-3: a review of its pharmacology, pharmacokinetics, and clinical use in transplantation. Pharmacotherapy 11(1):26-37.
- https://www.marketwatch.com/ press-release/monoclonal-antibody-therapy-market-2021-covid-19-impact-forecast-by-size-growth-rate-trends-share-business-insights-and-global-healthcare-sector-outlook-to-2026-2021-02-01.
- https://www.marketwatch.com/ press-release/small-molecule-drug-discovery-market-trend-survey-2021-with-top-countries-data-industry-growth-size-share-forecasts-analysis-company-profiles-competitive-landscape-and-key-regions-analysis-research-report-with-covid-19-analysis-2021-03-08.
- Philippidis A. Top fifteen best-selling selling drugs launched in 2020. Gen Eng News Retrieved 6th April, 2021, from https://www.genengnews.com/ a-lists/top-15-best-selling-drugs-launched-in-2020/.
- Romer T, Leonhardt H, Rothbauer U. (2011) Engineering antibodies and proteins for in vivo imaging. Curr Opin in Biotech. 22(6):882-887.
- Ecker DM, Jones SD, Levine HL. (2015) The therapeutic monoclonal antibody market. MAbs 7(1):9-14.
- Zhu G, Niu G, Chen X. (2015) Aptamer-drug conjugates. Bioconjugate Chem. 26:2186-2197.
- BrossPF, Beitz J, Chen G, Chen XH, Duffy E, Kieffer L, Roy S, Sridhara R, Rahman A, Williams G, Pazdur R. (2001) Approval summary: gemtuzumab ozogamicin in relapsed acute myeloid leukemia. Clin Cancer Res 7(6): 1490-1496.
- Davies SL, James DC (2009) Engineering Mammalian Cells for Recombinant Monoclonal Antibody Production. In: Al Rubeai M. ed. Cell engineering (Vol.6): Cell Line Development. Netherlands. Springer, 153-173.
- Maute RL, Gordon SR, Mayer AT, et al. (2015) Engineering high-affinity PD-1 variants for optimized immunotherapy and immuno-PET imaging. Proc. Natl. Acad. Sci. U.S.A. 112(47):E6506-14.
- Yoon S, Rossi JJ. (2018). Aptamers: Uptake mechanisms and intracellular applications. Adv. Drug Deliv. Rev. 134, 22–35.
- Aptamer Group. What is an aptamer? Retrieved 6th April 2021 from https://aptamergroup.com/what-is-an-aptamer/.
- Healy JM, Lewis SD, Kurz M, Boomer RM et al. (2004) Pharmacokinetics and biodistribution of novel aptamer compositions. Pharma Res. 21(12):2234-2246.
- Macugen.
- NOXXON Pharma. Pipeline. Retrieved on 6th April 2021 from https://www.noxxon.com/index.php?option=com_content&view=article&id=15&Itemid=503.
- Iveric Bio. Why Iveric? Retrieved on 6th April from https://ivericbio.com/why-iveric/.
- Rogers T, Bunka D. (2021) Synthesising new solutions for small molecule antibodies. Drug Discovery World Retrieved 19th April 2021 from https://www.ddw-online.com/synthesising-new-solutions-for-small-molecule-antibodies-10883-202104/ .
- Zhu G, Zheng J, Song E, Donovan M, et al. (2013) Self-assembled, aptamer-tethered DNA nano-trains for targeted transport pf molecular drugs in cancer theranostics. Proc. Natl. Acad. Sci. U.S.A. 110:7998-8003.
- Wang R, Zhu G, Mei L, Xie Y et al. (2014) Automated modular synthesis of aptamer-drug conjugates for targeted drug delivery. J. Am. Chem. Soc. 136:2731-2734.
- Han D, Zhu G, Wu C, et al (2013) Engineering a cell-surface aptamer circuit for targeted and amplified photodynamic cancer therapy. ACS Nano. 7:2312-2319.
- Cao Z, Tong R, Mishra A, Xu W et al. (2009) Reversible cell-specific drug delivery with aptamer-functionalized liposomes. Agnew. Chem., Int. Ed. 48:6494-6498.
- Li L, Hou J, Liu X, Guo Y, Wu Y, et al. (2014) Nucleolin-targeting liposomes guided by aptamer AS1411 for the delivery of siRNA for the treatment of malignant melanomas. Biomaterials 35(12):3840-50.
- Bagalkot V, Farokhzad OC, Langer R, Jon S. (2006) An aptamer-doxorubicin physical conjugate as a novel targeted drug-delivery platform. Agnew. Chem., Int. Ed.45:8149-8152.
- Dassie JP, Liu X, Thomas GS, Whitaker RM, et al. (2009) Systemic administration of optimized aptamer-siRNA chimeras promotes regression of PSMA-expressing tumors. Nat. Biotechnol. 27:839-846.
- Liang C, Guo B, Wu H, Shao N, Li D, et al. (2015) Aptamer-functionalized lipid nanoparticles targeting osteoblasts as a novel RNA-interference based bone anabolic strategy. Nat. Med. 21:288-294.
- Zhou J, Li H, Li S, Zaia J, Rossi JJ. (2008) Novel dual inhibitory function aptamer-siRNA delivery system for HIV-1 therapy. Mol. Ther. 16:1481-1489.
- Yoon S, Wu X, Armstrong B, Habib N, Rossi JJ. (2019) An RNA aptamer targeting the receptor tyrosine kinase PDGFRa induces anti-tumor effects through STAT3 and p53 in glioblastoma. Mol Ther Nucleic Acids 14:131-141.
- Macdonald J, Denoyer D, Henri J, Jamieson A, Burvenich IJG, et al. (2020) Bifunctional aptamer–doxorubicin conjugate crosses the blood–brain barrier and selectively delivers its payload to EpCAM-positive tumor cells. Nucleic Acid Ther. 30:117–128.
- Balasubramanyam J, Badrinarayanan L, Dhaka B, Gowda H, Pandey A, et al. (2019) EpCAM Aptamer siRNA chimeras: Therapeutic efficacy in epithelial cancer cells bioRxiv doi: https://doi.org/10.1101/656199.
- Kaur H, Bruno JG, Kumar A, Kumar Sharma T. (2018) Aptamers in the therapeutics and diagnostics pipelines. Theranostics. 8(15):4016-4032.
- Aptamer Group. Flow cytometry. Retrieved on 6th April 2021 from https://aptamergroup.com/flow-cytometry/.
- Yoon S, Rossi JJ. (2018). Aptamers: Uptake mechanisms and intracellular applications. Adv. Drug Deliv. Rev. 134, 22–35.
- Li X, Yang Y, Zhao H, Zhu T, Yang Z, et al. (2020) Enhanced in Vivo Blood–Brain Barrier Penetration by Circular Tau–Transferrin Receptor Bifunctional Aptamer for Tauopathy Therapy. J. Am. Chem. Soc. 142(8):3862-3872.
- Aptamer Group. Aptamer Group Announces Agreement with AstraZeneca to Explore Next-Generation Drug Delivery Devices. Retrieved on 6th April 2021 from https://aptamergroup.com/aptamer-group-announces-agreement-with-astrazeneca-to-explore-next-generation-drug-delivery-devices/.
- Aptamer Group. Aptamer Group extends collaboration with leading biopharmaceutical company to explore next-generation drug delivery approaches. Retrved on 6th April 2021 from https://aptamergroup.com/aptamer-group-extends-collaboration-with-leading-biopharmaceutical-company-to-explore-next-generation-drug-delivery-approaches/.To view this issue and all back issues online, please visit www.drug-dev.com.
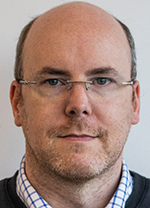
Dr. David Bunka is the CTO of Aptamer Group. He has spent over 20 years developing nucleic acid aptamers against a wide variety of targets, including small molecules, disease-associated proteins, several cancer-associated cell-lines, viruses and tissue biopsies. He earned his PhD in Molecular Biology from University of Leeds.
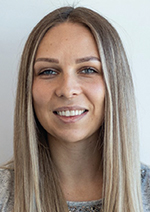
Dr. Emily Robinson leads the therapeutic development team at Aptamer Group to support early drug discovery for partners and collaborators. She has research experience in the evaluation of dysregulated signaling pathways in cancer and the identification of novel drug targets. She earned her PhD in Cancer Immunology from University of Leeds.
Total Page Views: 4809