Issue:June 2024
AMORPHOUS SOLID DISPERSION SPECIATION - Impact of Polymer Chemistry & Drug Properties
INTRODUCTION
Amorphous solid dispersions (ASDs) are the most successful formulation technology for oral delivery of poorly soluble compounds. ASDs, usually formed by spray drying, convert a crystalline form of a drug to an amorphous state. The amorphous state is a high-energy physical form that can enhance solubility beyond that of crystalline forms. In an ASD, API is typically formulated with a polymer that helps to both maintain the API in the amorphous form in the solid state and may act as a precipitation inhibitor to sustain the amorphous solubility upon dissolution.
Additionally, the congruent dissolution of API and polymer in the ASD particle can lead to the generation of solubilized drug-polymer colloids.1 Drug-polymer colloids can further enhance oral bioavailability by increasing the rate of diffusion of API, relative to undissolved solids, through the unstirred boundary layer to the surface of the intestinal epithelium. This can result in a rapid resupply of dissolved drug as API permeates through the epithelium.2
Formulation development of ASDs focuses on polymer selection, the ratio of drug to polymer, and the design of the ASD particles to develop a system that is physically stable while also demonstrating optimal performance. ASD performance is defined by enhancement in bioavailability, driven by supersaturation of dissolved drug and the formation of drug-polymer colloids. The following focuses on the use of in vitro techniques for characterizing ASD performance and on how these techniques can be used to help better understand the role of polymer chemistry in ASD performance.
BIORELEVANT DISSOLUTION STUDIES
The biorelevant dissolution study aims to evaluate the behavior of an API, drug product intermediate, or drug product under biorelevant conditions. Biorelevant conditions in these studies are defined primarily by the media composition and API concentration. The two most typical variations of this test are the “one-stage” and “two-stage” tests, referring to evaluation in simulated intestinal fluid or in simulated gastric fluid followed by a dilution into simulated intestinal fluid, respectively. These studies are often referred to as “non-sink” dissolution studies for low solubility compounds, as the target doses in vivo are typically well above the solubility of API in biorelevant media. For amorphous formulations, these studies are well-suited for assessing the potential for solubility enhancement relative to the crystalline API. Typical time courses may be from 3-24 hours and allow for assessment of the transient solubility enhancement that is typical of many amorphous systems.
A common dissolution procedure involves removing a sample from the dissolution vessel, centrifuging this sample using a microcentrifuge, and subsequently determining the concentration of drug in the supernatant. The material removed during centrifuging consists mostly of undissolved material suspended in the dissolution media. Simple measurements of the supernatant in the saturated, “non-sink”, solutions obtained in these studies quantitates the apparent solubility of the enabled formulation. This apparent solubility comprises dissolved drug, drug in bile-salt micelles (if included in the biorelevant media), and drug in drug-polymer colloids.3 These drug-containing “species” are useful when comparing formulations. However, additional insight into the speciation can be powerful toward understanding the performance of these formulations. For example, ultracentrifugation can be used to further separate these species. Ultracentrifugation of the supernatant separates the larger and denser drug-polymer colloids from the solvated free drug. The difference in drug concentration in the supernatant after microcentrifugation and ultracentrifugation represents the drug-polymer colloids. Due to the small size and low density of the drug-laden bile salt micelles, ultracentrifugation does not separate these species from freely dissolved (solvated) drug.
Figure 1 illustrates typical results from a single-stage biorelevant dissolution study for an ASD that demonstrates traditional “Spring & Parachute” behavior.4 In this system, there is an initial supersaturation (the “spring”) observed upon dissolution that is significantly above the crystalline solubility. In addition, this concentration of drug typically surpasses the amorphous solubility of the drug. The corresponding ultracentrifuge data demonstrates supersaturation above the crystalline solubility but does not surpass the amorphous solubility. The amorphous solubility is the highest possible solubility the free solubilized drug can achieve in a given media – any apparent solubility measurements that surpass this value are attributable to solubilized species. The difference in the microcentrifuge and ultracentrifuge values thus represents the presence of drug-polymer colloids. Supersaturation is transient, and precipitation to crystalline solubility will eventually occur. The length of time supersaturation is sustained (the “parachute”) is determined by the various drug interactions with micelles, polymers, and other excipients and endogenous material in vivo.
Figure 2 illustrates results from a single-stage biorelevant dissolution study for an ASD that demonstrates amorphous sustainment rather than the “Spring & Parachute” motif. This behavior, for which the conversion to a crystalline form is exceedingly slow, is common for larger and/or more complex API modalities, such as bifunctional protein degraders and peptides. In this system, the ASD dissolves in an aqueous media to the inherent amorphous solubility, demonstrating significant enhancement over the crystalline API. In many cases, this effect occurs for drug alone, with no excipients, such as polymers, present. However, in most cases, the use of excipients can significantly enhance dissolution rate, and may also provide additional drug species that can transit through the GI tract efficiently. Drug-polymer colloids are evidenced by the difference between the micro- and ultracentrifuged values. However, unlike the “Spring & Parachute” system, the amorphous solubility is sustained within the time frame relevant to this study and to GI transit, and precipitation to crystalline solubility is not observed.
THE EFFECTS OF POLYMER CHEMISTRY
The polymer in an ASD serves several critical functions. In the solid state, the polymer stabilizes the amorphous dispersion by providing a separation of drug molecules and significantly reduced diffusion. A surrogate measurement of diffusion is the glass transition temperature of the dispersion. It is imperative diffusion in the solid state is managed appropriately so the risk of crystallization of the amorphous drug is extremely low. The polymer can also impact powder properties, which may be critical for downstream processing to enable the incorporation of the ASD into an oral solid dosage form. The polymer also impacts performance through the rate of dissolution, acting as a precipitation inhibitor to sustain supersaturation, and by forming drug-polymer colloids that can further enhance oral bioavailability.
Polymer chemistry can impact supersaturation and the propensity for the formation of drug-polymer colloids in a variety of ways.5 In general, the hydrophilicity of a polymer influences the final behavior of an ASD that incorporates the polymer. Typically, more hydrophilic polymers tend to produce polymer colloids that are smaller and less dense, which can aid in initial supersaturation, but can lead to faster precipitation for API that exhibit strong crystallization tendencies. Conversely, more hydrophobic polymers tend to produce larger, more dense colloids that can slowly release drug molecules into solution and can serve to promote sustainment of the amorphous solubility over longer periods of time. However, the effects these differences in colloidal properties have on API solubilization are also influenced by the strength of the interaction between a given API and polymer, as well as their relative dissolution rates and physicochemical properties of the API. Thus, it is important to screen these performance metrics by investigating a range of polymers.
Figure 3 illustrates the use of a two-stage biorelevant dissolution study to evaluate the effect of polymer chemistry on supersaturation and the formation of drug-polymer colloids. These experiments demonstrated a clear pattern indicating that enteric or more hydrophobic polymers generally demonstrate the best sustainment profiles, with HPMCAS-H, despite the slower dissolution rate, providing greater sustainment in dissolved drug and the formation of drug-polymer colloids when compared with HPMCAS-M.
FLUX TESTING
While non-sink dissolution experiments are useful for evaluating the extent of solubilization and colloidal speciation for ASD formulations, they do not directly indicate the tendency of those formulations to promote absorption of the drug. To evaluate the ability of the species formed by ASDs to promote permeation across the intestinal epithelium, in vitro flux testing can be useful.6 Flux experiments utilize a semi-permeable membrane that is coated in phospholipids that is placed between two chambers – the donor and receiver chambers. In the donor chamber, a two-stage non-sink dissolution experiment is performed, and the concentration of API is evaluated in both the donor and receiver chamber. By comparing the solubilization and sustainment of the API in the donor chamber, and the extent of diffusion into the receiver chamber, we can assess the general tendency of ASD formulations to promote API permeation in vivo.
Figure 4 shows results from an in vitro flux experiment that demonstrates the effect of ASD formulation on both supersaturation and permeation. In this experiment, the amount of drug loading in the ASD formulation is indirectly correlated with the extent of supersaturation and permeation, with the 50% HPMCAS-M formulation achieving higher concentrations in both the donor and acceptor chambers. These results indicate this formulation may promote both supersaturation and absorption of the drug.
SUMMARY
With the rise in use of ASDs to enable improved in vivo absorption of poorly soluble compounds, it is important to be able to evaluate performance in vitro to understand the effect of formulation on in vivo performance. The use of non-sink dissolution methods to quantify the apparent solubility of enabled formulations is a valuable approach for selection of the preferred formulations. By leveraging ultracentrifugation to better understand how speciation, influenced by drug behavior and polymer chemistry, enhances supersaturation, additional insights into formulation selection can be achieved. Additionally, in vitro flux testing can be useful to understand the ability of enabled formulations to promote permeation across the intestinal epithelium. A thorough understanding of the application of these methods and a deep understanding of the impact of excipient selection on performance, physical stability, processability, and dosage form performance is critical to optimize the development of ASDs.
REFERENCES
- Anura S. Indulkar et al., “Insights into the Dissolution Mechanism of Ritonavir-Copovidone Amorphous Solid Dispersions: Importance of Congruent Release for Enhanced Performance,” Molecular Pharmaceutics 16, no. 3 (2019): 1327–39, https://doi.org/10.1021/acs.molpharmaceut.8b01261.
- Aaron M. Stewart et al., “Impact of Drug-Rich Colloids of Itraconazole and HPMCAS on Membrane Flux in Vitro and Oral Bioavailability in Rats,” Molecular Pharmaceutics 14, no. 7 (2017): 2437–49, https://doi.org/10.1021/acs.molpharmaceut.7b00338.
- Dwayne T Friesen et al., “Hydroxypropyl Methylcellulose Acetate Succinate-Based Spray-Dried Dispersions: An Overview,” Molecular Pharmaceutics 5, no. 6 (December 1, 2008): 1003–19, https://doi.org/ 10.1021/mp8000793.
- Yanbin Huang and Wei-Guo Dai, “Fundamental Aspects of Solid Dispersion Technology for Poorly Soluble Drugs,” Acta Pharmaceutica Sinica B 4, no. 1 (2014): 18–25, https://doi.org/10.1016/j.apsb.2013.11.001.
- David E. Alonzo et al., “Dissolution and Precipitation Behavior of Amorphous Solid Dispersions,” Journal of Pharmaceutical Sciences 100, no. 8 (2011): 3316–31, https://doi.org/10.1002/jps.22579; Huang and Dai, “Fundamental Aspects of Solid Dispersion Technology for Poorly Soluble Drugs”; Indulkar et al., “Insights into the Dissolution Mechanism of Ritonavir-Copovidone Amorphous Solid Dispersions: Importance of Congruent Release for Enhanced Performance”; Hajime Konno et al., “Effect of Polymer Type on the Dissolution Profile of Amorphous Solid Dispersions Containing Felodipine,” European Journal of Pharmaceutics and Biopharmaceutics 70, no. 2 (October 2008): 493–99, https://doi.org/10.1016/j.ejpb.2008.05.023.
- Akshay Narula, Rayan Sabra, and Na Li, “Mechanisms and Extent of Enhanced Passive Permeation by Colloidal Drug Particles,” Molecular Pharmaceutics 19, no. 9 (2022): 3085–99, https://doi.org/10.1021/acs.molpharmaceut.2c00124.
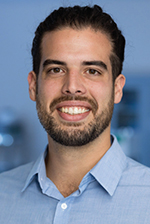
Dr. Wesley Tatum is a Principal Engineer in the Process and Product Development department at Serán. He leads a team of scientists and engineers in characterizing new API and identifying shortcomings for bioavailability. He and his team then screen and select drug product intermediates that address those shortcomings and screen and scale up drug product formulations. During his time at Serán, his work and research has focused on understanding how polymers, excipients, and physiological factors influence speciation, bioavailability, and absorption of drug substances, and amorphous solid dispersions. He earned undergraduate degrees in Physics and Physical Chemistry from Whitworth University and his PhD in Materials Science and Engineering from the University of Washington.
Total Page Views: 4096