Issue:June 2013
LIPOSOME PREPARATION TECHNOLOGY - Freeze Fracture Electron Microscopic (ff EM) Examination & Analysis of Liposomes Produced by Covaris AFA Technology
INTRODUCTION
Recently, there has been considerable renewed interest in technologies to form liposomes. Although there are few commercialized liposomal products, there are many in various stages of clinical development.1,2 The reason for the renewed interest is that both poorly soluble APIs and difficult-to-deliver biological molecules like proteins, peptides, and genes can potentially be more readily formulated as liposomes to both increase biological uptake and improve effectiveness of treatment. This potential quicker formulation would accelerate more efficient and more cost-effective candidate screening.
The traditional liposome preparation methods, including detergent depletion, ethanol injection, reverse-phase evaporation, and emulsion methods, all have intrinsic limitations. Depending on the moieties to be packaged and the desired mechanism of action, liposomes can be prepared in three forms: Multi Lamellar Vesicles (MLV), Large Unilamellar Vesicles (LUV), and Small Unilamellar Vesicles (SUV). MLVs have a more complex structure than LUVs and SUVs.1-3 Processing methods include high-pressure homogenization, extrusion, and traditional ultrasound. The major disadvantages of these preparation methods include the requirement for significant volumes of volatile organic solvents and their subsequent solvent evaporation, multiple lengthy processing steps, contamination, and potential degradation of the samples due to heat exposure.3-5 These limitations can be problematic for the delivery of some types of active materials, most notably solvent- or temperature-sensitive biological compounds.
Traditional ultrasound, which is unfocused and low frequency, has been in use for preparing liposomes for many years.1 However, it has fundamental limitations as a process technology due to inefficient energy conversion and poor control to obtain a desired pressure flux, and the inherent long wavelength precludes effective process control. An alternative ultrasonic processing technology, based on Covaris’ Adaptive Focused AcousticsTM (AFA), allows manufacturing of liposomes of predetermined sizes and offers an efficient, isothermal, and non-contact process.
Formation of liposomes using AFA has been described in previous articles.3 AFA utilizes a concave transducer to converge emitted acoustic energy to a precise point inside a processing vessel without direct contact. This enables processing vessels to be loaded with liposome-forming materials and buffers and simultaneously exposed to an active acoustic focal point. This allows control of cavitation and streaming energy to form liposomes of particular sizes. Temperature of the sample is maintained by immersion in a water bath, which is connected to a chiller with a preset temperature. The AFA process employs focused bursts of ultrasonic acoustic energy at frequencies 20 to 40 times higher than traditional sonicators. The high frequency of AFA produces a wavelength of only a few millimeters, which enables the ultrasonic acoustic energy to be focused into a discrete zone within a sample vessel immersed in a water bath. AFA Focusedultrasonicators are highly controllable and may be independently programmed for the power intensity, duration, and duty factor of these bursts. The versatility in power settings supports a wide variety of applications, from low-power gentle mixing and enzyme acceleration, to high-power DNA shearing, tissue disruption, and compound management, including dissolution, formulation, and emulsification.
AFA technology enables a simple and efficient liposome preparation workflow, and provides effective thermal control with no cross-contamination when compared to other available liposome preparation technologies.3-8
In this article, blank liposomes were produced using AFA technology, followed by characterization using Dynamic Light Scattering (DLS) and ff EM (freeze fracture Electron Microscopy) techniques. Although in previous articles, the particle size distribution of liposomes was analyzed using DLS, a more detailed confirmation of liposome structure is required.3 ff EM techniques are widely used to assess the surface morphology of liposome formation and provide a more detailed evaluation of liposome construction using AFA.
Three different lipid (liposome) formulation samples were prepared and formed. To evaluate structure of liposomes formed below phase transition temperatures, the third liposome sample was prepared using DSPC (1,2-distearoylsn- glycero-3-phosphocholine), which has a phase transition temperature above 55°C (Tc of DSPC is 55°C to 58°C).9 This liposome sample was formed upon chilling the phospholipid to 3°C and maintaining the sample temperatures below 20°C during preparation. Critical questions of the Covaris liposome process include:
1. Can AFA liposomes be prepared without organic solvents?
2. Is the particle size of the AFA liposomes as small as the liposomes prepared using traditional methods?
3. What is the structure of AFA liposomes formed at temperatures below the gel-to-liquid crystalline phase transition temperature (Tc) of the lipid?
MATERIALS
Phospholipon 90G (EP-80) by Lipoid (Newark, NJ); Doxil lipids, ie, HSPC [L-a- phosphatidylcholine, hydrogenated (Soy)], DSPE-PEG2000 [1,2-distearoyl-sn-glycero -3- phosphoethanolamine -N- [methoxy(polyethyleneglycol) -2000] (ammonium salt)], and DSPC (1,2- distearoyl-sn-glycero-3-phosphocholine) from Avanti lipids (Alabaster, AL), cholesterol and 0.1M Phosphate-buffered Saline (PBS) from Sigma (St Louis, MO); Covaris focused-ultrasonicator Model S220x in closed 2-ml sample processing vessels (PN 520056) both from Covaris, Inc. of Woburn, MA.
PREPARATION OF AFA LIPOSOMES
The respective lipids were weighed and added to 2-ml glass vessels. Sample 1 was processed for 12 mins using the S220x acoustic settings of 75-Watt Peak Incident Power (PIP), 50% Duty Factor (DF), and 1000 Cycles per Burst (C/B). Samples 2 and 3 were processed for 1 hr using the S220x acoustical settings of 150-Watt PIP, 50% DF, and 1000 C/B. 20 mg/ml o phospholipon 90 G is used in Sample 1. In Sample 2, 11.4 mg HSPC was used with 4.5 mg of PEG DSPE and 4 mg of cholesterol. In Sample 3, 20 mg/ml of DSPC was used. Respective lipids were washed into process vessel and topped off with 0.1M PBS, followed by tightening the vessel cap and loading into the S220x for processing at 3°C chiller temperature.
PREPARATION PROCEDURE (NANOANALYTICAL LABORATORY STANDARD TEST METHOD STM – 001)
The ff EM was performed by Nano Analytical Laboratory (San Francisco, CA). The samples were quenched using the sandwich technique and liquid nitrogencooled propane. Using this technique, cryofixation at a cooling rate of 10,000°C/sec is reached, and ice crystal formation and artifacts possibly caused by the cryofixation process are minimized. The cryofixed samples were stored in liquid nitrogen for less than 2 hrs before processing. The fracturing process was carried out in JEOL JED-9000 freezeetching equipment, and the exposed fracture planes were shadowed with Platinum for 30 secs in an angle of 25 to 35 degrees and with carbon for 35 secs (2kV/ 60 to 70 mA, 1×10-5 Torr). The replicas produced this way were cleaned with concentrated, fuming nitric acid for 24 hrs followed by repeating agitation with fresh chloroform at least 5 times. The replicas were examined using a JEOL 100 CX electron microscope. Multiple micrograph images were taken for each sample. Information on the evaluated samples is provided in Table 1, and images are shown in Figures 1 through 3.
RESULTS
Sample 1 – EP-80 Liposomes
DLS measurement analysis is presented in Table 2. Figure 1 shows the surface morphology by ff EM analysis, and Table 1 represents the sizing analysis by ff EM. The particle average diameter (Zaverage) as measured by DLS is 360 nm, while ff EM analysis shows it to be around 170 nm. Budding of smaller liposomes from larger sizes is observed. A significant number of smaller particles are found. These EM pictures show MLV, LUV, and SUV and their transformation from one shape to another, which suggests that the sample may be only partially processed, and additional processing may result in more complete convergence on a specific size distribution.
Sample 2 – Doxil Liposomes
DLS measurement analysis is presented in Table 2. Figure 2 shows the surface morphology by ff EM analysis, and Table 1 represents the sizing analysis by EM. The Z-average as measured by DLS is 142 nm, while ff EM analysis shows it to be around 90 nm. Even here, bilayer particles were found. Particles had agglomerated due to storage conditions (4°C) before performing the EM analysis.
Sample 3 – DSPC Liposome
DLS measurement analysis is presented in Table 2. Figure 3 shows the surface morphology by ff EM analysis, and Table 1 represents the sizing analysis by EM. The Z-average as measured by DLS is 198 nm, while ff EM analysis shows it to be around 30 nm.
DISCUSSION
Electron micrographs taken from several freeze-fracture preparations show all three samples contain overall spherical particles in high concentrations. Because most of these particles display their shadows mainly in front and behind their structures, they showed bilayer-coated structures such as liposomes.
The average diameter of these liposomes is largest at 170 nm in Sample 1 (EP-80), followed by the diameter in Sample 2 (Doxil Lipids) at 90 nm, and the smallest diameter in Sample 3 (DSPC) of 30 nm. The size range of the particles is very large in the EP-80 sample (from 20 nm to 2.3 micrometers) and smaller in the Doxil lipids sample (from 15 to 640 nm) as well as the DSPC sample (from 9 nm to 1.2 micrometers). Size ranges in Samples 2 and 3 may read higher due to agglomeration, which results in DLS representing a group of particles as a single entity (especially in Sample 2). The observed distribution of particles matches the polydispersity index (PdI) values obtained by DLS analysis. This value for EP-80, Doxil, and DSPC liposomes were 0.514, 0.390, and 0.276, respectively. Agglomeration in the 3 samples was observed. As reported in the literature, this agglomeration occurred primarily due to the 4°C sample storage condition.8-10 In addition, agglomeration could have benefited from dilution before characterizing with ff EM.
In Samples 1 and 2, liposomes consist of several bilayers (MLVs). The particles in Sample 3 are mostly unilamellar (SUVs), although they are at the lower end of the particle size of SUVs. In Sample 3, some particles that are even smaller than the smallest liposomes are visible (9 to 14 nm), showing shadows mostly behind their structures, which may well represent phospholipid micelles. This finding is not surprising and most likely due to the low processing temperature used in preparing liposomes that include a phospholipid that has a high phase transition temperature (Tc = 54°C) in which the lipid bilayer is in the gel state.
In addition to these observations, every sample exhibited some special characteristics. Smaller liposomes seem to “bud-off ” from the most outer bilayers of larger liposomes (Figure 1). AFA process with EP-80 at low power produced liposomes that have more than one bilayer (multiple layers). This would be useful in increasing the loading of entrapped API. Liposomes formed are circular in shape. This shows that first, larger particles are formed and then the smaller liposomes bud off from the large ones. Based on previous liposome processing using AFA, if this sample was processed for only 2 to 3 mins, larger particles would result. Likewise, if the sample was processed for more than 12 mins (ie, 24 mins or longer), smaller particles would be formed, and the number of bilayers and size of vesicles would most likely decrease.
Most of the liposomes appeared edgy, such as seen clearly in Samples 2 & 3. Even though the Doxil liposomes are agglomerated particles, the Z-average is around 90 nm, which is near the reported particle size of 87.5 nm for Doxil formulation.7
Small DSPC liposomes (around 30 nm) are produced by AFA. This confirms that liposomes can be formed below the phase transition temperature of DSPC (Tc = 54°C) because the chiller temperature was maintained at 3°C, and the sample temperature was measured to be between 16°C to 20°C. Most of the particles are single or represent small-numbered associates (Sample 3). There are few areas visible where these small particles are not totally separated from larger units (Figure 3). Sample 3 supports the concept of forming liposomes with the bulk fluid temperature below Tc.
CONCLUSION
AFA-based formation of liposomes is confirmed in all three samples. Indeed, even in the DSPC sample processed below the phase transition, liposomes were formed. Overall, there was a fairly good agreement in the sizing data (Z-average values) between DLS and EM for the EP-80 and Doxil liposomes. For the DSPC liposomes, a large discrepancy between the two methods was observed.
By simply changing the process conditions of AFA, both the size and distribution of the liposome population can be changed. Preparation methods are simple and convenient, and offer dramatic improvements over current methods. For example, the elimination of solvents and subsequent rehydration of the lipid offers considerable time savings over existing methods.
In conclusion, the AFA technology offers a viable new delivery system method for potentially formulating biologically active liposomes, especially for temperature- and solvent-sensitive molecules.
REFERENCES
1. Torchilin VP. Recent advances with liposomes as pharmaceutical carriers. Nat Rev Drug Discov. 2005;4(2):145-160.
2. Whitehead KA, Langer R, Anderson DG. Knocking down barriers: advances in siRNA delivery. Nat Rev Drug Discov.2009;8:129-138.
3. Kakumanu S, Schroeder A. Focused ultrasound – a novel tool for liposome formulation. Drug Development & Delivery. 2012;12:47-52.
4. Kakumanu S, Bernhard J. Adaptive Focused Acoustics for the formulations of suspensions & nanosuspensions. Drug Development & Delivery. 2011;11:36-41.
5. Tejera-Garcia R, Sanjeev R, Vladimir Z, Rohit S, Paavo KJK. Making unilamellar liposomes using focused ultrasound. Langmuir. 2011;27(16):10088-10097.
6. Volinsky R, Lukasz C, Piotr J, Martin H, Pavel J, Paavo KJK. Oxidized phosphatidylcholines facilitate phospholipid flip-flop in liposomes. Biophys J. 2011;101(6):1376-1384.
7. Sanjeev R, Rohit S, Jozsef D, et al. Peptide-mediated targeting of liposomes to TrkB receptor-expressing cells. Int J Nanomedicine. 2012;7:3475-3485.
8. Jing Z, Ya Z, Weikai Z, et al. Preclinical nanomedicine: internalization of liposome nanoparticles functionalized with TrkB ligand in rat cochlear cell population. Eur J Nanomed. 2009;3:7- 13.
9. Saarinen-Savolainen P, Jarvinen T, Taipale H, Urtti A. Method for evaluating drug release from liposomes in sink conditions. Int J Pharm.1997;159:27-33.
10. Bao A, Goins B, Klipper R, Negrete G, Phillips WT. Direct 99mTc labeling of pegylated liposomal doxorubicin (Doxil) for pharmacokinetic and non-invasive imaging studies. J Pharmacol Exp Ther. 2003;308(2):419-425.
11. Frank BH, Ira WL. Lipid vesicle aggregation induced by cooling. Int J Mol Sci. 2010;11:754-761.
12. Lis LJ, Mcalister M, Fuller N, Rand RP. Interactions between neutral phospholipid bilayer membranes. Biophys J. 1982;37:657-666.
13. Larrabee AL. Time-dependent changes in the size distribution of Distearoyl phosphatidyl choline vesicles. Biochem.1979;18(15):3321-3326.
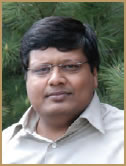
Dr. Srikanth Kakumanu earned his PhD from the Department of Biomedical Engineering and Biotechnology at University of Massachusetts in 2010. Since June 2010, he has been working as a Research Scientist at Covaris, Inc., where he heads the research in the application of Adaptive Focused Acoustics in formulations (Dissolution, Micronization, Nanosuspensions, and Liposomes). His major focus of research is scaling the AFA process to pilot scale and continuous flow processing.
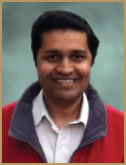
Dr. Rajeev A Jain joined Covaris, Inc. in 2013 as Manager of Business Development – Formulations. Dr. Jain has more than 15 years of broad experience in various drug delivery technologies, formulation/process development, technology transfer, scale-up, and product outsourcing. Previously, he has held scientific and technical positions at Lonza Biologics, Fleet Laboratories, Acusphere Inc., and Elan Drug Technologies. Dr. Jain has authored or co-authored a variety of abstracts and publications. Over 8 patents and patent applications have been issued or filed under his name. Dr. Jain earned his MS in Pharmacognosy and his PhD in Pharmaceutics from The University of Rhode Island.
Total Page Views: 3135