Issue:November/December 2013
API INTEGRATION - Modification of Silicone Chemistry & Its Influence on Release Rates of APIs
INTRODUCTION
Silicones are well known and continually used the world over for their biological compatibility and chemical stability.1 The most researched biomaterials to date, they have been a trusted component of medical device applications since the 1950s, and popularity of their use has only increased with advancements in technology. Silicones can be found in cochlear implants, joint orthopedics, birth control devices, and pacemakers – to name a few common uses. Considering the expansiveness of the healthcare field, perhaps the most advantageous feature of silicone is that it can be designed to achieve specific performance, aesthetic, or therapeutic properties not inherently offered by relatively basic silicone formulations. Silicone is so versatile that its properties can vary greatly and even contradict each other, from one silicone to the next. For instance, basic polydimethylsiloxane (PDMS) silicone is inherently insulative at approximately 1015 Ω∙cm, but with the addition of certain fillers, a silicone can be made to conduct heat, electricity, or both.
A decade after silicone’s auspicious advent into the medical arena, this versatile, synthetic material was first used for the controlled release of drugs, or Active Pharmaceutical Ingredients (APIs), into the body – an employment it has retained to the present time.2 Commercial drug delivery devices that incorporate silicone are commonly manufactured for the following purposes: contraception, hormonal replacement, and infection mitigation with antibiotics.
Integrating APIs into a silicone system requires consideration of multiple factors, such as compatibility of the silicone with the API and, more generally, achievement of the prescribed dose delivery rate over the prescribed amount of time. Because different APIs can potentially alter the properties of silicone and vice versa, modification of silicone chemistry may be necessary.3
SILICONE CHEMISTRY
Comprising the siloxane polymer backbone of a silicone are repeating helical silicon-oxygen bonds (Si-O), with substituent, or R’, groups attached to the open valences of the silicon atom. Compared to epoxies and other organic-based rubbers with Carbon-Carbon (C-C) bonds, the Si-O bonds of silicones provide larger bond angles.1 These bond angles yield large amounts of free volume, leaving space for design or, more specifically, for managing the amount and type of substituent group and filler, including APIs, that go into a silicone system. This ability for formulation flexibility is largely what enables silicone to be so versatile.
Varying the substituent groups on the polymer chain can help optimize silicones for very specific needs. These components interact with the Si-O-Si units to cause the resulting silicone material to exhibit certain properties. Common R’ groups that commend silicone for use in the medical field are methyl, phenyl, and trifluoropropyl (fluoro). Methyl, formative of polydimethylsiloxane polymers (PDMS), is most known for its water resistance and desirable surface properties, which aid in moisture retention and contribute to lubricity and gentleness in topical or more intrusive applications. This is the most common substituent group found in biomedical applications. Moreover, phenyl groups can be employed in resulting diphenyldimethylpolysiloxane polymers to increase or decrease a silicone’s permeability to moisture as needed, as well as to adjust its refractive index, or RI. As in the case of wound care dressings, external prosthetic devices, and contact lenses, silicone often needs to be permeable in order to act as a membrane through which water can be transmitted to surrounding tissue, and optical clarity is frequently required of protective encapsulants for assessment of components. Fluoro imparts swell resistance to silicone materials, which can be susceptible to size increase when in contact with common solvents, or other acidic fluids, such as stomach acids.
In addition to rendering the substituent groups on the polymer interchangeable, allowing for control of mechanical and physical properties, free volume is also one of the reasons silicone is so often made a conduit for the delivery of various actives to particular parts of the body. The other reason is silicone’s chemical stability for its role in preserving the active’s effectiveness at no cost to the silicone’s material integrity or properties. Drug delivery applications are some of the most complex in the medical world; chemical stability is dependent upon the goals and parameters at hand. Thus, marrying a silicone formulation to a drug profile virtually always involves trial and error.
EXPERIMENTAL SECTION: BACKGROUND
NuSil Technology conducted a study through which the antibiotics rifampin and minocycline were to be incorporated into a silicone matrix and completely released in equal portions at a steady rate by the end of 14 days.
Rifampin is best known for use against bacteria that causes tuberculosis. The human body (generally speaking) develops resistance to rifampin quickly, so the drug is partnered with one or more antibiotics for functional assistance. Rifampin’s companion in this case study, minocycline, may be used to treat certain strains of MRSA infections, amoebic dysentery, cholera, and pneumonia. This broad spectrum tetracycline antibiotic is classified as a long-acting type and so complements rifampin for coexistence in the same device.
For medical device simulation, both APIs were eluted through a silicone molded component. Due to the interaction of the APIs with the silicone chemistry being a potential concern, the appropriate silicone system needed to be identified. The appropriate system would also be one that accommodated ease in processing and molding, key for effectiveness in its final form as a vehicle for eluting the APIs. Among the most common silicone types in the healthcare field are gels and elastomers.
Silicone gels are made of reactive silicone polymers and reactive silicone crosslinkers in a two-part system that yields little to no elastomeric strength. When cured, these low-viscosity materials are designed to have a soft, compliant feel which, combined with silicone’s low modulus, allows them to mimic human tissue. They range from very soft for prosthetic applications to very tacky (sticky) for topical and transdermal wound care applications. Encapsulation of electronics, such as for use in certain implantable sensors, is also a common application for using silicone gels.
Silicone elastomers are similar in composition to gels but exhibit increased physical and mechanical properties due to high levels of reinforcing fillers and longer polymer chains. For instance, elastomers have higher viscosities than gels and fluids. High consistency rubbers (HCRs) are ideal for extruded tubing because their silica-reinforced, high molecular weight polymers enable them to maintain a shape when uncured; low consistency elastomers (LCEs), by contrast, are flowable and more ideal for coatings, encapsulants, and molded parts requiring optical clarity. Compared to HCRs and liquid silicone rubbers (LSRs), the high clarity and low viscosity associated with LCEs are primarily attributed to their unique base formulations, which may incorporate phenyl. Of intermediate viscosity, LSRs are used to mold high precision parts, such as gaskets, valves, O-rings, and seals. HCRs, LCEs, and LSRs are moldable materials that can be cast or injected into molds of various configurations.
Over gels, different silicone rubbers were chosen to begin the case study for their chemical makeup and consistency, imparting greater material integrity. The chosen system was modified throughout the trials so as to yield the elution results desired without compromising the mechanical strength of the silicone.
EXPERIMENTAL SECTION: RESULTS
Before the release rates of rifampin and minocycline were measured, a screening test was conducted to ensure the silicone would cure after incorporation of the APIs. This is a vital first step when incorporating any new API into a silicone system. The result may determine if additional formulating is necessary to counteract the effects caused by the API’s interaction with the silicone, or if a different cure chemistry is required due to compatibility concerns. In addition, an exhaustive extraction test verified the APIs were not chemically bonding with the silicone matrix. Via elution testing, the release rates of rifampin and minocycline were tested with comprehensive evaluation a total of four times.
First attempt: An LCE, a liquid fluorosilicone rubber, a high durometer HCR, and a low durometer HCR were selected for the first elution test. The first elution test showed that per each formulation, the APIs were not being released at a sufficient rate to yield full elution after 14 days. Of the silicone materials tested, the fluorosilicone released the highest amounts of minocycline and rifampin. Further testing on the silicones and the APIs revealed that high temperatures made the actives no longer antimicrobial. To equalize the release rates and maintain the integrity of the actives, three new versions of the low durometer HCR were developed for a second elution test. To enable cure at a lower temperature, a fugitive inhibitor in the original fluorosilicone formulation was replaced with a competitive inhibitor. Inhibitors are formulary ingredients utilized to control and regulate working times (pot life) of the blended elastomer as well as their cure profiles (rate of cure at a specified temperature).
Second attempt: After some formulary adjustments were made to the silicones used in the first attempt, three low durometer HCRs were used for the second elution test. A noticeable improvement to the first trial results, the release rates of the second trial showed greater elution of the actives. However, the pharma-grade excipients intended to maximize elution diminished the physical properties of the system and produced a product difficult to process. The adjusted silicone systems were either too tacky or not tacky enough for processing on the two-roll mill. Formulary changes made after these results anticipated process-friendly material and retention of uniform release rates.
Third attempt: Again, additional formulary adjustments were made in an attempt to produce better elution results. Six new formulations of the low durometer HCR were developed for the third elution test. Six formulations of low durometer HCRs were developed based on results of the second elution test. For better processing performance of the silicone, additional pharma-grade excipients were selected. Results showed that with these excipients, the silicone matrix could be more easily processed, and that some combinations promoted equivalent release of rifampin and minocycline – though no formulations fully eluted the drugs in 14 days. On some of the formulations, additional optimization required to achieve full release degraded the cured properties of the silicone. Adjustments made for the next formulation were designed to maintain the processing performance achieved in the third trial, preserve the mechanical properties of the silicone, and elude the APIs equally in 14 days.
Fourth attempt: Formulation 7 from the third attempt was redeveloped and optimized for what would be the last elution. The parameters of the study were met on the fourth trial. As is evident from Figure 5, release of the actives was uniform and occurred within the desired time frame of 14 days. Further testing showed that the cure profile for the silicone matrix was met, its mechanical integrity maintained, and its consistency compatible for two-roll mill processing.
CONCLUSION
The results discussed herein reiterate that (1) silicone chemistry lends itself to optimization and (2) formulation modification influences the release of actives. What modifications are needed for the success of drug delivery applications are dependent upon the applications’ parameters and goals, including but not exclusive to the following: temperature limitations, inhibition concerns, cure profile, desired rheology, the meeting of elution profiles, and the maintenance of the physical integrity of the vulcanized silicone system. The increase in the number and kind of drug delivery applications has furthered silicone technology for the controlled incorporation and elution of APIs like rifampin and minocycline. Within the scope of assaying the release of APIs through a silicone matrix, over 100 elution studies were executed and the materials involved evaluated for mechanical, elution, and processing performance. Advancements in silicone technology are born from studies such as the one presented herein, thus augmenting through customization the ability of silicone components to meet and sustain the needs of drug delivery applications.
REFERENCES
1. Abbasi F, Mirzadeh H, Katbab A. Modification of polysiloxane polymers for biomedical applications: a review. Polymer Int. 2001;50:1279-1287.
2. Kurnellas A, Wolfe N. Understanding the role of silicones in controlled release applications. Medical Design Technol. June 17, 2010. Website visited: http://www.mdtmag.com/articles/2010/06/understanding -role-silicones controlled-release-applications.
3. Leonard S. Injecting new ideas into active drug delivery systems. Qmed. April, 2008. Website visited: http://www.qmed.com/mpmn/article/silicones-show-its-okay-be-passive.
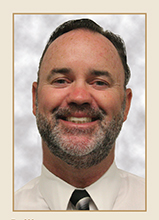
Brian Reilly is the Marketing & Sales Director for Medical Implants at NuSil Technology LLC. He earned his BS in Biological Sciences from Cal Poly San Luis Obispo before being hired by NuSil, where he has worked for over 15 years. Mr. Reilly began his career in the R&D department formulating and developing silicone elastomers and adhesives. From there, he moved into his current role in Marketing & Sales.
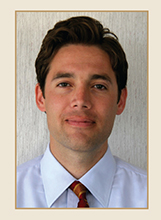
Nathan Wolfe is a NuSil Technology Technical Sales Representative and Drug Delivery Market Specialist who began his 14-year career at NuSil Technology in its Regulatory Affairs department, where he supervised regulatory submissions to the FDA and oversaw contract review. As the Market Specialist for drug delivery applications, he is responsible for NuSil Technology’s new Drug Delivery Silicones (DDS) Product Line as well as NuSil’s expansion into targeted release and combination product applications.
Total Page Views: 2164