Issue:January/February 2015
EXCIPIENT UPDATE - Soluplus®: An Understanding of Supersaturation From Amorphous Solid Dispersions
Amorphous Solid Dispersions (ASDs) have been widely accepted as a desired solution for enhancing solubility and bioavailability of poorly soluble drugs.1 With a trend in increased number of poorly soluble new chemical entities (NCEs), the industry is adapting non-conventional formulation technologies, exploiting the existing polymers, and exploring innovative ones to expedite drug development.2 However, the applicability of those polymers in such technologies has also created new challenges associated with processing and manufacturing or thermal instability, such as hot melt extrusion and Kinetisol®, or residual solvents, such as spray drying and co-precipitation.3-6
A number of polymers, including polyvinylpyrrolidone (PVP), copovidone (PVP-VA), hydroxypropylmethylcellulose (HPMC), hydropropylmethylcellulose acetate succinate (HPMCAS), amino methacrylate copolymer among others have been used in ASD formulations.1c However, a clear understanding of factors responsible for maintaining supersaturation and stability of drug molecules to overcome precipitation and retardation of dissolution in the gastrointestinal milieu, and their impacts on the bioavailability of APIs from polymeric ASDs, is still lacking and thus highly warranted.7-10
Soluplus®, a polyethylene glycol, polyvinyl acetate and polyvinylcaprolactame-based graft copolymer (PVAc-PVCap- PEG), has been studied extensively in ASDs of several investigational, and model drugs in hot melt extrusion,11-21 spray drying,22-24 high shear dispersions, Kinetisol,25 electrospinning/electrospraying,26-27 microwave radiation,28-29 solvent casting,30 solvent evaporation,31-33 ball milling,34 physical/co-milling blends,35-36 and thermal heating38 amongst others.
This article is aimed at understanding the in vitro solubilization of a model drug CBZ, more specifically, at the interplay between achieved supersaturation and flux of a model drug from Soluplus amorphous dispersions. The mechanisms underlying the supersaturation and diffusive flux of drug across membrane from ASDs will also be discussed and postulated.
MATERIALS & METHODS
Soluplus (BASF, Florham Park, NJ) was used as received. Carbamazepine was extruded with Soluplus on a 16-mm twin screw extruder (Thermo Fisher) under the processing conditions as reported previously.38 A miniature device μFLUX™ together with in situ fiber optic dissolution monitoring system μDISS Profiler™ (Pion, MA, Figure 1) were used to measure flux of free drug in receiver compartment separated from the donor by the artificial membrane.39
RESULTS
Solubilization of CBZ & CBZ-Soluplus Extrudates: Dissolution of CBZ & CBZ Soluplus Dispersions in SGF & FaSSIF
Figures 2A and 2C illustrate the dissolution and solubility of pure crystalline CBZ in SGF (pH 1.2) and FaSSIF (pH 6.5) buffers. It is clear that as the dissolution progressed, the CBZ concentration peaked in the first 30 min reaching approx. 200 μg/ml. As the dissolution continued, the solubility of CBZ declined due to re-crystallization equilibrating at ~150 μg/ml, and stayed constant for > 16 hours.40 With Soluplus dispersions, on the other hand, CBZ solubility increased and peaked at >300 μg/ml, and stayed in supersaturation for over 16 hours without precipitation in both SGF and FaSSIF media (Figures 2B and 2D). The solubility of CBZ in ASDs was at least 2-fold higher than that in pure CBZ and was also independent of pH changes. Because the ASD of CBZ was fully dissolved in this experiment, the solubility enhancement ratio could not be determined.
Dissolution & Flux of CBZ at pH 7.4
To further explore the supersaturation, the solubility of CBZ was investigated using 2-chamber μFLUX system. The donor compartment contained pure drug or ASD formulation (1mg/mL CBZ load in pH 7.4 Prisma HT buffer), while the receiver chamber contained pH 7.4 buffer and a surfactant to simulate the sink conditions.39 Figures 3A and 3B show the concentration – time profile of CBZ in donor and receiver chambers, respectively. The CBZ concentration continued to increase in the receiver with flux changing slightly depending on the concentration level in the donor.
In Soluplus dispersion, CBZ peaked to maximum concentration of 1 mg/ml, maintaining the supersaturation over 4 hours (240 min) as illustrated in Figure 4A. It ultimately followed a gradual precipitation, but the CBZ flux in the receiver chamber continued to stay nearly constant despite precipitation of CBZ in the donor (Figure 4B).
The data suggests that flux of free CBZ in the receiver from Soluplus dispersions was about 3-fold higher than that from pure CBZ at pH 7.4 in over 4 hours. The higher concentration of CBZ in donor resulted in higher flux of CBZ across the membrane in receiver during all phases; dissolution, supersaturation, and precipitation of drug.
Figure 5 illustrates curves from dissolution, supersaturation, and precipitation processes during the first 200 min from pure CBZ (Figure 3B) and Soluplus dispersions (Figure 4B) at pH 7.4 ASB buffer. Figure 5 illustrates that despite quick re-precipitation of CBZ, it was possible to detect differences between the initial flux of 1.2 μg-min-1•cm-2 (supersaturation region), the flux during precipitation (0.92 μg•min-1•cm-2), and the flux when the concentration of CBZ reached equilibrium concentration (0.85 μg•min-1•cm-2). The flux from CBZ-Soluplus remained nearly constant within a 0.5- to 3-hour period and was ~3 times higher than for pure CBZ. Following the onset of re-precipitation, it decreased only slightly from 2.7 to 2.4 μg•min-1•cm-2.
DISCUSSION
This study describes the application of Soluplus, a hydrophilic polymeric solubilizer, in solid dispersions of a model drug carbamazepine. The scope of this study is limited to understanding of hydrophilic polymers and their behaviors on amorphous dispersions. Therefore, the studies from other hydrophilic polymers have been examined in the context of drug’s solubility, loading, precipitation, dissolution, and supersaturation, and also compared with Soluplus dispersions. Such comparisons are necessary to help identify the polymers based on a postulated model for achieving the desired supersaturation.
Amorphous Solid Dispersions With Hydrophilic Polymers
Sun and Lee studied the effects of infusion of a hydrophilic polymer (PVP K-90) on supersaturation of model drugs in amorphous dispersions.41 The gradual infusion of the crystalline inhibitor PVP increased the maximum indomethacin concentration and attained the supersaturation much longer as opposed to a faster infusion, suggesting that the hydration of PVP was critical for achieving and maintaining a higher kinetic solubility. Like PVP, hydrophilic polymers, such as HPMC, were also effective and maintained the supersaturation of felodipine and nifedipine from amorphous dispersions.42
Verreck and Brewster observed that the addition of hydrophilic polymers PVP (K-30) and PEG 400 did not help maintain the supersaturation of an investigation compound (m.p. 300°C), but the addition of polyoxyl hydrogenated 40 castor oil (Kolliphor® RH40) with PVP K-30 (1:3), resulted in maintaining the supersaturation.43 Addition of Poloxamer 407 in Soluplus dispersions with carbamazepine not only helped facilitate the extrusion process but also improved the loading and miscibility of drug in the polymer, and hence, increased the dissolution rate.12 In a recent study, vitamin E TPGS (Kolliphor® TPGS) when used in copovidone (PVPVA) extrudates inhibited the precipitation and increased the miscibility and loading of carbamazepine and fenofibrate (unpublished).
Intermolecular interactions and complexation originating from Hbonding, ionic, and/or van der Waal’s interactions of hydrophilic polymers with drugs play an important role in solubilization, stability, and maintaining supersaturation.44 For example, Soluplus with multiple interaction sites increased the solubility of albendazole as high as 50% in amorphous dispersions also maintaining the supersaturation, while, HPMCAS neither enhanced solubility nor resulted in supersaturation. With other model drugs, such as fenofibrate, the HPMCAS performed better than Soluplus and/or HPMCE5 and maintained supersaturation, and significantly improved the bioavailability in rats.45 In other cases, HPMCAS performed relatively better than copovidone with itraconazole dispersions, presumably due interactions between an alkaline drug and an acidic polymer,46 while it performed sluggish as compared with Soluplus dispersion.23 In cases where drugs are sensitive to acidic or alkaline, changing the microenvironment pH or counter-ions of ASD might be equally important for preventing re-crystallization and achieving the supersaturation, and increasing the kinetic solubility insolution.21 In a recent study, inclusion of Soluplus in atorvastatin calcium dispersions also prevented recrystallization, and achieved 3.6-fold higher bioavailability in rats from amorphous dispersions as compared to physical mixtures.22 In our study, solubilization of CBZ from a physical mixture was identical to Soluplus amorphous dispersion, suggesting that drug was likely solubilized in the polymeric micelles, which prevented recrystallization and maintained the supersaturation (data not shown). Thus, the lipophilic and solubilizing characteristics of Soluplus are crucial for complexing with drugs to help not only for maintaining supersaturation in vitro but also increasing the bioavailability.15,47
Qian et al observed that the solubility and loading of an investigation compound (BMS-A) was significantly higher in PVP-VA, which led to faster recrystallization and slower dissolution and lower bioavailability compared to HPMCAS.48 In a study with Soluplus containing 15% itraconazole, the extrudates were stable over 3 months under accelerated conditions and did not show any signs of surface recrystallization or retardation of dissolution (unpublished). The stability of ASDs was also dependent upon the processes used in manufacturing. For example, when Soluplus, HPMCAS and PVP were used in spray drying and melt extrusion of felodipine, the dissolution rates were comparable for both spray dried powders and melt extrudates, but the physical stability of the extrudates was better than the spray dried powders due, in part, to stronger interactions of drug and polymer caused by intimate mixing at higher processing temperatures.11 The presence of residual solvents in ASD powders caused phase separation and formation of local drug domains. Soluplus dispersions with 15% itraconazole prepared by melt extrusion, and spray dried also behaved identically. Both formulations maintained supersaturation for an extended period before re-crystallization.23
Solubilizers also play an important role in influencing the permeation of drugs across the membranes from solid dispersions. Kanzer et al examined the effects of sorbitan monolaurate, polyoxyl 40 hydrogenated castor oil and propylene glycol laurate on permeation of calcein through phospholipid barrier from the melt extrudates composed of copovidone (PVA-VA) copolymer as a placebo and with two HIV drugs.49 The surfactants with lower HLB values, for example, propylene glycol and sorbitan monolaurate (ca. HLB 4-6) did not show any change in permeability and electrical resistance, and hence, were both compatible with lipid barrier. In contrast, polyoxyl 40 hydrogenated castor oil (HLB 16) in both API free and API containing melt extrudates increased permeation of calcein primarily attributed to leakage of the membrane. This is consistent with an earlier study wherein polyoxyl 35 castor oil (HLB 12-14) was also incompatible, and caused the leakage of lipid membrane.50 Whentested with CaCo2 cells, the PVPVA/drug dispersions containing sorbitan monolaurate, the flux of drugs was much higher as compared to lipid barrier, suggesting that active and passive transports both interplayed for such increase.53
Raina et al examined the flux of felodipine and nifedipine through a cellulosic membrane (MWCO of 6-8 K) with HPMC as a crystalline inhibitor in aqueous solution. The flux of drugs increased linearly in relation to donor concentration as long as drugs remained in the supersaturation.42 The data from our studies also suggests that the flux of CBZ through PAMPA membrane from Soluplus dispersions was 2.5- to 3-fold higher than flux from untreated CBZ.51
Mechanisms Underlying the Supersaturation
The data from the Soluplus and carbamazepine study, and the examples cited in this article from the literature with hydrophilic polymers, shed light on understanding of supersaturation from amorphous solid dispersions. It also highlights the factors responsible for drug and polymer interactions and stability of amorphous dispersions, to help identify the physico-chemical characteristics of polymers relevant to supersaturation. Hence, three possible scenarios are proposed to further simplify the supersaturation phenomenon, and are illustrated in Figure 6.
1. Curve 6A may arise from polymers possessing good solubilization and crystalline inhibitory properties. The combination of both attributes could lead to increased kinetic solubility and maintaining supersaturation, and will appear as a parachute before precipitation. Maintaining such behavior could lead to significantly higher in vitro trans-membrane flux and meet narrow therapeutic windows for the absorption and bioavailability of drugs.
2. Curve 6B may arise from polymers possessing some degrees of solubilization but probably lacking a significantly larger lipophilic characteristic, thereby, limiting the complexation with drugs. In such cases, the desired solubility of drugs can be achieved, but supersaturation will be maintained briefly yielding a limited kinetic solubility and exhibiting a “spring” behavior, before the precipitation begins. This trend could be reversed to a parachute Curve A by the addition of a surfactant or solubilizer with higher HLB values, and/or a hydrophilic polymer with desired crystalline inhibitory properties.
3. Curve 6C may arise from polymers possessing moderate to poor solubilization and complexation abilities, wherein, the supersaturation could hardly be achieved due to precipitation (limited kinetic solubility), leading to an immediate drop in the dissolution upon exposure to aqueous solutions. This trend could be reversed by the addition of an appropriate solubilizer and/or polymers with desired crystalline inhibitory properties, and in such cases, Curve C could follow the same trend as Curve B with limited kinetic solubility, or in a rare case, will follow the Curve A, allowing to maintain the supersaturation for an extended period.
CONCLUSIONS
Soluplus offers an advantage over many other polymers to study the supersaturation phenomenon due, in part, to its inherent amphiphilic characteristics derived from lipophilic and hydrophilic polymeric components. The data from this study clearly demonstrates that Soluplus possesses all the physicochemical attributes for improving solubilization, maintaining supersaturation, and preventing the recrystallization of drugs. Our data demonstrates that Soluplus dispersions maintains carbamazepine concentrations at least 3-fold higher than pure crystalline drug and also maintains the supersaturation like the Curve A (Figure 6). The outstanding in vitro performance of Soluplus dispersions could also help understand the increased in vivo performances of the extrudates of other model drugs in rats and beagle dogs.15,22
The study showed that the increase of flux of CBZ in the receiver compartment is lower than the increase in apparent kinetic solubility of the drug from CBZ-Soluplus ASD, suggesting that the dissolved CBZ is present in both unbound form (free drug) and CBZ-Soluplus complex (bound form) in donor compartment. Thus, the dissolution permeability μFLUX™ device can be used to simultaneously monitor the flux of free drugs in solutions from a complex solid dispersion system. Additional studies are necessary at molecular levels to understand the supersaturation and its correlation with thermodynamic and kinetic stability and flux of drugs in the aqueous and biorelevant solutions.
To view this issue and all back issues online, please visit www.drug-dev.com.
REFERENCES
1. (a) F. Qian, J. Huang, and M. A. Hussain, Drug-polymer solubility and miscibility: Stability consideration and practical challenges in amorphous solid dispersion development, J. Pharm. Sci., 2010, DOI 10.1002/jps.22074; (b) S. B. Teja, S. P. Patil, G. Shete, S. Patel, and A. K. Bansal, drug-excipient behavior in polymeric amorphous solid dispersions, J. Excipients & Food Chem., 2013, 4, 70-94; (c) C. L-N. Vo, C. Park, and B-J. Lee, Current trends and future perspectives of solid dispersions containing poorly water-soluble drugs, Eur. J. Pharm. Biopharm., 2013, 85, 799-813.
2. (a) D. E. Alonzo, G. G. Z. Zhang, D. Zhou, Y. Gao, and L. S. Tylor, Understanding the behavior of amorphous pharmaceutical systems during dissolution, Pharm. Res., 2009, 27, 608-618; (b) A. S. Narang, R-K. Chang, and M. A. Hussain, Pharmaceutical development and regulatory considerations for nanoparticles and nanoparticulate drug delivery systems, J. Pharm. Sci., DOI 10.1002/jps.23691.
3. J. L. Santos, P. Cordeiro, and M. Temtem, Scale-up of spray dried amorphous solid dispersions, Eur. Indust. Pharm. 2013, 19, 4-8.
4. B. Li, M. He, W. Li, Z. Luo, Y. Guo, Y. Li, C. Zang, B. Wang, F. Li, S. Li and P. Ji, Dissolution and pharmacokinetics of baicalin–polyvinylpyrrolidone coprecipitate, J. Pharm. Pharmacol., 2013, 65, 1670-1678.
5. J. R. Hughey, J. M. Kean, D. A. Miller, C. Brough, J. W. McGinity, Preparation and characterization of fusion processed solid dispersions containing a viscous thermally labile polymeric carrier, Int. J. Pharm. 2012, 438, 11-19.
6. D. A. Miller, J. C. DeNunzio, J. R. Hughey, R. O. Williams III, and J. W. McGinity, Kinetisol: A new processing paradigm for amorphous solid dispersion system, Drug Development & Delivery, 2012, 30-39.
7. N. Kohri, Y. Yamayoshi, H. Xin, K. Iseki, N. Sato, S. Todo, K. Miyazaki, Improving the oral bioavailability of albendazole in rabbits by solid dispersion technique, J. Pharm. Pharmacol., 1999, 51, 159-164.
8. N. Newa, K. H. Bhandari, D. X. Li, T. Kwon, J. A. Kim, B. K. Yoo, J. S. Woo, W. S. Lyoo, C. S. Yong, H. G. Choi, Preparation, characterization and in vivo evaluation of ibuprofen binary solid dispersions with poloxamer 188, Int. J. Pharm. 2007, 343, 228-237.
9. (a) Six et al., Clinical study of solid dispersions of itraconazole prepared by hot stage extrusion, Eur. J. Pharm. Sci., 2005, 24, 179-186; (b). K. Six, G. Verreck, J. Peeters, M. Brewster, and G. V. den Mooter, Increased physical stability and improved dissolution properties of itraconazole, a Class II drug, by solid dispersions that combine fast- and slow-dissolving polymers, J. Pharm. Sci., 2004, 93, 124-131.
10. N. Shah, R. M. Iyer, H-J. Mair, D. S. Choi, H. Tian, R. Diodone, K. Fahnrich, A. Pabst-Ravot, K. Tang, E. Scheubel, J. F. Grippo, S. A. Moreira, Z. Go, J. Mousakountakis, T. Louie, P. N. Ibrahim, H. Sandhu, L. Rubia, H. Chokshi, D. Singhal, and W. Malick, Improved human bioavailability of vemurafenib, a practically insoluble drug, using an amorphous polymerstabilized solid dispersion prepared by a solvent controlled co-precipitation, J. Pharm. Sci., 2013, 102, 967-981.
11. Y. Tian, V. Caron, D. S. Jones, A-M. Healy, and G. P. Andrews, Using Flory-Huggins phase diagrams as a preformulation tool for the production of amorphous solid dispersions: a comparison between hot-melt extrusion and spray drying, J. Pharm. Pharmacol., 2013, 66, 256-274.
12. J. Djuris, N. Ionnis, S. Ibric, Z. Djuric and K. Kachrimanis, Effect of composition in the development of carbamazepine hot melt extruded solid dispersions by application of mixture experimental design, J. Pharm. Pharmacol., 2013, 66, 232-243.
13. I. Durate, M. Temtem, J. F. Pinto, M. Gil and F. Gaspar, Screening methodology for the development of amorphous solid dispersions, PBP World Meeting.
14. L. Sambath, A. K. Mathu, M. A. Kumar, K. Phaneendra, and Shalini, Physicochemical characterization and in vitro dissolution behavior of gliclazide-Soluplus solid dispersions, Int. J. Pharm. Pharmaceutical Sci., 2013, 5, 204-210.
15. M. Linn, E-M. Collnot, D. Djuric, K. Hempel, E. Fabian, K. Kolter, and C-M. Lehr, Soluplus as an effective absorption enhancer of poorly soluble drugs in vitro and in vivo, Eur. J. Pharm. Sci., 2012, 45, 336-343.
16. R. Rajeswari and A. K. Sudhakar, Development, characterization and solubility study of solid dispersion of valsartan, J. Chem. Pharm. Res. 2011, 3, 180-187.
17. R. Fule and P. Amin, Development and evaluation of lafuthidine solid dispersion via hot melt extrusion: Investigating drug-polymer miscibility with advanced characterization, Asian J. Pharm. Sci., 2014, 9, 92-106.
18. R. A. Fule, T. S. Meer, A. R. Sav, and P. D. Amin, Artether-Soluplus hot melt extrudate solid dispersion systems for solubility and dissolution rate enhancement with amorphous state characteristics, J. Pharm., Volume 2013, Article ID 151432, 15 pages.
19. A. K. Mehata, V. Suryadevara, S. R. Lankapalli, A. M. Deshmukh, and L. P. Sambath, Indonesian J. Pharm., 2013, 24, 206-214.
20. S. Chavan, K. Patel, D. Shelar, and P. Vavia, Preparation of oxcarbazepine solid dispersion by hot melt extrusion for enhanced dissolution: downstream processing to tablets, Am. J. Pharm. Res., 2013, 3, 557-569.
21. C. Kulkarni, A. Kelly, T. Gough, and A. Paradkar, Stability study of artemisinin-Soluplus solid dispersion (www. Pharmaceutical-engineering.brad.ac.uk).
22. E-S. Ha, I-H. Baek, W. cho, S-J. Hwang, and M-S. Kim, Preparation and evalulation of solid dispersion of atorvastatin calcium with Soluplus® by spray drying technique, Chem. Pharm. Bull., 2014, 62, 545-551.
23. D. Smithey, J. Fennewald, J. Gautschi, M. Crew, S. Ali, Y. Lan, and N. Langley, evaluation of the polymer Soluplus for spray dried dispersions of poorly soluble compounds, AAPS poster 2010.
24. B. Rashmika, V. Veena, S. Kachhwaha, and D. V. R. N. Bhikshapathi, Der Pharmacia Lettre, 2013, 5, 73-82.
25. R. Hughey, J. M. Keen, D. A. Miller, K. Kolter, N. Langley, and J. W. McGinity, The use of inorganic salts to improve the dissolution characteristics of tablets containing Soluplus-based solid dispersions, Eur. J. Pharm. Sci., 2013, 48, 758-766.
26. U. Paaver, I. Tamm, I. Laidmäe, A. Lust, K. Kirsimae, P. Veski, K. Kogermänn, and J. Hein ämääki, Soluplus graft copolymer- Potential novel carrier polymer in electrospinning of nanofibrous delivery systems for wounds therapy, Biomed. Res. International, 2014,2014, 1-7.
27. Z. K. Nagy, A. Balogh, B. Vajna, A. Farkas, G. Patyi, A. Kramarics, and G. Marosi, Comparison of electrospun and extruded Soluplus- based solid dosage forms of improved dissolution, J. Pharm. Sci, 2011; DOI 10.1002/jps.22731.
28. M. Sharma, R. Garg, and G. D. Gupta, Formulation and development of solid dispersion of atorvastatin calcium, J. Pharm. Sci. Innovation, 2013, 2, 73-81.
29. A. Hussain, I. Ermolina, N. I. Bhkhari, K. A. Khan, and G. Smith, Milling and co-milling with various excipients for the improvement of intrinsic dissolution rate of ibuprofen, UKPharmSci, 2013, Edinburgh, Sept. 2-4.
30. M. M. Pandey, G. K. Kumar, R. Ramakrishan and S. Chardet, Solubility enhancement of felodipine by solid dispersions with novel polymeric solubilizer Soluplus, J. Bioequi. Availab, 2012, 4(3), 146.
31. A. Homayouni, F. Sadeghi, J. Varhosaz, and H. Garekani, Soluplus: a novel excipient to improve dissolution rate of poorly water soluble drug, celecoxib, Res. Pharm. Sci., 2012, 7(5), S386.
32. G. Jyothirmal and V. V. N. Rao, Solubility enhancement of gliclazide by solid dispersion technique, Int. J. Inv. Pharm. Sci., 2013, 1, 542-553.
33. C. Mendiratta, V. Kadam, and V. Pakharkar, Lansoprazole solid dispersion using a novel amphiphilic polymer Soluplus, J. Chem. Pharm. Res., 2011, 3, 536-543.
34. V. Caron, Y. Hu, L. Tajber, A. Erxleben, O. I. Corrigan, P. McArdle, and A. M. Healy, Amorphous solid dispersions of sulfonamide/Soluplus and sulfonamide/PVP prepared by ball milling, AAPS PharmSciTech, 2013, 14, 464-474.
35. K. R. Shankar and K. P. R. Chowdary, Formulation development of efavirenz tablets employing b-cyclodextrin, Soluplus and PVP K30: factorial study, Int. Res. J. Pharm. App. Sci., 2013, 3, 110-115.
36. N. A. Khetrapal, A. R. Sav, L. Rao, and P. D. Amin, Formulation development of stable solid oral dosage form of valproic acid using colloidal silica, Int. J. Drug Del., 2012, 4, 266-274.
37. G. Vamkas, Q. Fang and A. Fatmi, Improved dissolution profile of API BCS Class IV drugs using solubility enhancement technologies, AAPS Poster 2011.
38. H. Hardung, D. Djuric, and S. Ali, Combining HME and solubilization: Soluplus® –The solid solution, Drug Del. Tech., 2010, 10, 20-27.
39. (a) A. Avdeef, and O. Tsinman, PAMPA -A drug absorption in vitro model 13. Chemical selectivity due to membrane hydrogen bonding: In combo comparisons of HDM-,DOPC-, and DS-PAMPA models, Eur. J. Pharm. Sci., 2006, 28, 43-50; (b). A Avdeef, Absorption and Drug Development. Solubility, Permeability and Charge State. John Wiley&Sons. 2012.
40. K. Tsinman, O. Tsinman, N. Langley, and S. Ali, Soluplus maintains the supersaturation of carbamazepine from amorphous solid dispersions, AAPS poster, 2012.
41. D. D. Sun and P. I. Lee, Evolution of supersaturation of amorphous pharmaceuticals: The effect of rate of supersaturation generation, Mol. Pharm. 2013, 10, 4330-4346.
42. S. A. Raina, G. G. Z. Zhang, D. E. Alonzo, J. Wu, D. Zhu, N. D. Carton, Y. Gao, and L S. Tylor, Enhancements and limits in drug membrane transport using supersaturated solutions of poorly soluble drugs, J. Pharm. Sci., 2013; DOI 10.1002/jps.23826.
43. G. Verreck, J. Peeters, and M. Brewster, Dissolution optimization and solid dispersion feasibility approaches for an anti-HIV drug candidate, CRS Poster, 2014.
44. (a) L. A. Wegiel, L. J. Mauer, K. J. Edger, ad L. Tylor, Midinfrared spectroscopy as a polymer selection tool for formulating amorphous solid dispersions, J. Pharm. Pharmacol., 2013, 66, 244-255; (b) Y. Li, H. Pang, Z. Guo, L. Lin, Y. Dong, G. Li, M. Lu and C. Wu, Interactions between drugs and polymers influencing hot melt extrusion, J. Pharm. Pharmacol, 2013, 66, 148-166.
45. M. Zhang, H. Li, B. Lang, K. O’Donnell, H. Zhang, Z. Wang, Y. Dong, C. Wu, and R. O. William III, Formulation and delivery of improved amorphous fenofibrate solid dispersions prepared by thin film freezing, Eur. J. Pharm. Biopharm., 2012, 82, 534-544.
46. P. Gao, B. D. Rush, W. P. Pfund, T. Huang, J. M. Bauer, W. Morozowich, M. S. Kuo, and M J. Hageman, Development of a supersaturable SEDDS (S-SEDDS) formulation of paclitaxel with improved bioavailability, J. Pharm. Sci., 2003, 92, 2386-2398.
47. S. Ali, N. Langley, D. Djuric and K. Kolter, Soluplus, Tablets & Capsules – Eye on Excipients, 2010, 8, #7.
48. F. Qian , J. Wang, R. Hartley, J. Tao, R. Haddadin, N. Mathias, and M. Hussain, Solution behavior of PVP–VA and HPMC–AS-based amorphous solid dispersions and their bioavailability implications. Pharm Res 2012, 29,2765–2776.
49. J. Kanzer, I. Tho, G. E. Flaten, M. Magerlein, P. Holig, G. Fricker, and M. Brandl, In-vitro permeability screening of melt extrudate formulations containing poorly water-soluble drug compounds using the phospholipid vesicle-based barrier, J. Pharm. Pharmacol., 2010, 62, 1591-1598.
50. G. E. Flaten, K. Luthman, T. Vasskog, and M. Brandl, Drug permeability across a phospholipid vesicle-based barrier: 4. The effects of tensides, co-solvents and pH changes on barrier integrity and on drug permeability, Eur. J. Pharm. Sci., 2008, 34, 173-180.
51. K. Tsinman and O. Tsinman, Dissolution-permeability apparatus with integrated in situ concentration monitoring of both donor and received compartments, AAPS Poster 2013.
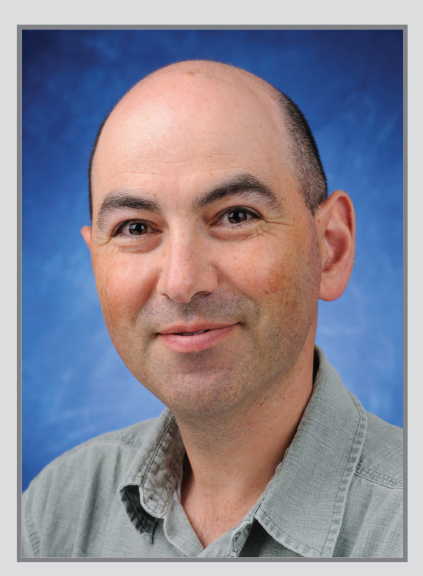
Ms. Oksana Tsinman is a Senior Scientist and a Manager of the Research and Analytical Laboratory at Pion Inc. She earned her Master’s in Biochemistry in the Ukraine in 1990. Ms. Tsinman joined Pion in 2002 and quickly became an integral part of Pion’s research team working on R&D projects that included among others optimization of high throughput solubility-permeability measurements, developing early stage formulation screening techniques and applying in situ UV fiber-optic measurements to preformulation screening. Her research in artificial membrane permeability measurements that would predict blood-brain-barrier transport was a basis for Pion receiving Phase I and II grant from the NIH. Her experimental skills and attention to the details made her a key part of multiple collaborative research projects with scientists from the pharmaceutical industry and academic institutions. She has co-authored more than 15 articles presented at international conferences and published in primary scientific journals.
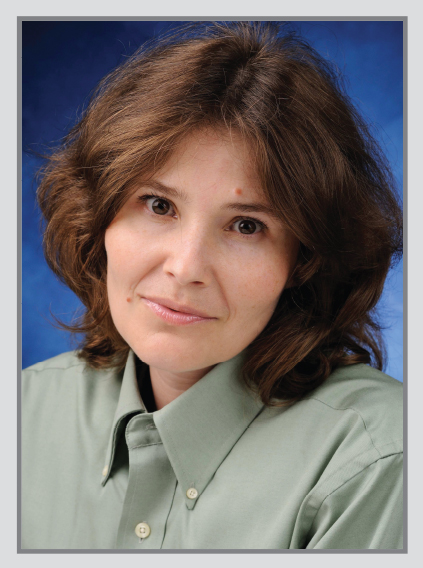
Dr. Konstantin Tsinman is the Director of Science and Research at Pion Inc. He joined the company in 1998 as principal developer of the very first commercial Parallel Artificial Membrane Permeability Assay (PAMPA) instrument and subsequently the high-throughput method for measuring solubility – pH profiles. He has been participating in numerous studies expanding the scope of applications for in situ UV fiber-optic instrumentation and utilization of derivative spectroscopy techniques for real-time concentration analysis of complex formulations in turbid solutions. Dr. Tsinman has been involved in multiple collaborative research projects with scientists from the pharmaceutical industry and academic institutions. He has co-authored more than 25 articles published in primary scientific journals and holds several patents. He earned his PhD in Physics in 1994 from the Institute for Metal Physics, Kiev, Ukraine.
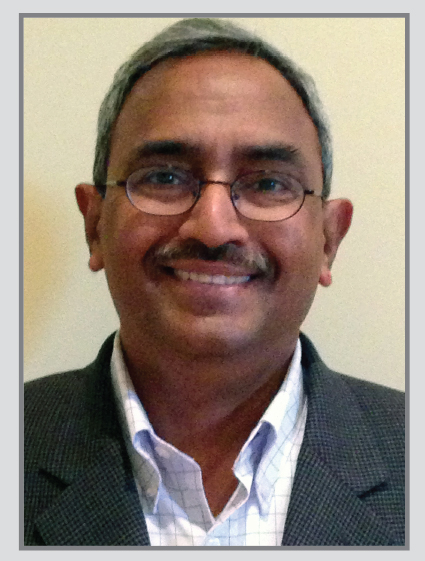
Dr. Shaukat Ali has over 20 years of experience in the pharmaceutical industry, including 10 years at BASF, where he supports the solubilization platform and APIs. He serves the USP panel of experts for General Chapters-Physical Analysis. He is also a member of the editorial boards of American Pharmaceutical reviews, Contract Pharma, Drug Development & Delivery, Biopharma Asia (UK), and International Journal of Pharmaceutical Investigation. He has authored over 25 scientific articles and is inventor/co-inventor in 14 US patents. He earned his PhD in Chemistry from the City University of New York and pursued his postdoctoral interest at the University of Minnesota and Cornell University. Dr. Ali’s areas of expertise include drug solubilization, liposome drug delivery, controlled release, and film development technologies.
Total Page Views: 13592