Issue:July/August 2016
PHARMACOLOGY MODELS - Early Phase Pharmacodynamic Models for Respiratory Drug Candidates
INTRODUCTION
In the past few years, the prevalence of respiratory diseases has grown significantly, and now about half a billion people around the world are thought to be affected. Furthermore, the mortality rate has doubled in the past 40 years, in stark contrast to other conditions, such as cardiovascular disease, cancer, and infections.1
The most common respiratory diseases are asthma and chronic obstructive pulmonary disease (COPD). Their increased prevalence can be attributed to an increase in exposure to common risk factors, and the ageing population.
Inhaled therapies predominate in treatment, and two different therapeutic agents are often given in combination. Advances in treatment are, for the most part, the result of better insights into the mechanisms that cause disease, particularly the role of inflammation. These insights have also resulted in the potential for phenotyping and endotyping to inform treatment. The phenotype represents those clinical features that are determined by a combination of the genotype and the environment, while the endotype represents the subtypes defined by distinct physiological mechanisms.
The pharmacodynamic techniques and surrogate outcome parameters that are used in developing new treatments for respiratory disease, however, lack sensitivity for testing new drugs. Several primary outcome measurements are commonly used in clinical trials in the respiratory field. These include dynamic lung volume after 1 second of forced expiration (FEV1), clinical exacerbations, and a variety of more subjective scales that measure impaired health and perceived wellbeing, such as the St George respiratory questionnaire (SGRQ), which is used in the study of COPD. These metrics all lack the required levels of sensitivity, and there is a poor correlation between the measurements and overall survival.2
There is a clear need for more effective and meaningful techniques across the board. These could help by reducing the number of patients who need to be included in trials, and the resulting increased efficiency of early development should lead to a lower attrition rate in confirmatory studies. Several new alternatives are already being used in clinical practice, but have yet to make the leap into drug development.
BODY PLETHYSMOGRAPHY
Spirometry is the standard method for measuring most relative lung volumes; however, it is incapable of providing information about absolute volumes of air in the lung. Thus, a different approach is required to measure residual volume, functional residual capacity, and total lung capacity. The technique of body plethysmography, also referred to as “body box,” is increasingly used in clinical practice because it is better controlled and enables a greater number of more sensitive dynamic lung volumes to be made than with FEV1 or vital capacity. It is also capable of measuring other parameters, such as residual volume, oscillometry, diffusion capacity for carbon monoxide, and airway resistance. Further advantages lie in using the box for testing reversibility of bronchoconstriction after administration of a bronchodilator, and its ability to offer more repeatable bronchial challenge tests. If used in clinical studies, it has the potential to give more sensitive measurements.
CHALLENGE METHODS
In clinical development, respiratory challenge tests allow proof-of-concept studies to be carried out in healthy volunteers, and also in patients who are only mildly to moderately ill. A number of different challenge tests are possible, including bronchoprovocation testing.
This test has been used for many years, and relies on the inhalation of increasing concentrations of a histamine solution and the responsiveness of the airway being measured, and can be used for the diagnosis and quantification of bronchial hyperreactivity. The test is commonly used in clinical practice for the diagnosis of asthma, and can replace or supplement reversibility testing, a protocol that is notorious for the number of false negatives it generates in well-controlled mild-to-moderate asthma. The test can also be used in COPD, specifically asthma-COPD overlap syndrome (ACOS). If it is used in clinical trials, it can increase recruitment potential, and thus decrease the screen failure rate. The test is also used in some reimbursement criteria.
The measurement used in these studies is PC20, ie, the concentration of challenge agent that produces a 20% reduction in FEV1. However, there are drawbacks. Histamine causes a number of adverse reactions, including headache, tachycardia, and bronchoconstriction, which makes it difficult to use successfully. There are alternatives with fewer drawbacks, which are used less frequently. These include metacholine, which exerts its action via the direct stimulation of bronchial smooth muscle cells, and adenosine, which has an indirect effect as it causes mast cell degranulation, which releases proinflammatory mediators. All three agents – histamine, methacholine, and adenosine – can be used for early proof-of-concept trials for either bronchoprotection or bronchodilation studies, largely in asthma.
For allergen testing, conditions have to be even more rigorously controlled to ensure exposure to external seasonal allergens is negated. Recently, the use of a mobile chamber was presented that could challenge up to nine patients within an optimal testing environment.3
Challenge tests can also be undertaken using inhaled lipopolysaccharide (LPS). This endotoxin is a toll-like receptor 4 (TLR4) agonist that activates cytokine production. It invokes an acute inflammatory response in the lung, which is one of the important mechanisms in play in asthma and COPD, and thus, can be used in the study of antiinflammatory drugs in these diseases. The endotoxin is administered via inhalation using an ultrasonic nebulizer, with a dose of up to 50 μg/ml of isotonic saline, or as an intravenous infusion of 4 ng/kg over a 2-minute period for other indications such as sepsis. Its effects are measured in induced sputum via the number of granulocytes, or the levels of various cytokines, such as TNF-alpha, IL-1beta, IL-6, IL-8, and IL-10.
As an example, in a Phase I single and multiple ascending dose proof-of-concept study in asthma, an LPS challenge was carried out on two cohorts, one consisting of healthy male subjects, and the other asthmatic patients. The 50-μg/ml dose of endotoxin was inhaled over a 2-minute period via five deep breaths of the nebulized solution. Sputum induction was carried out at pre-set time points after the challenge using increasing concentrations of hypertonic saline, up to 5%.
The viral challenge model, meanwhile, can give high-quality proof-of-concept safety and efficacy data, notably in upper respiratory tract infections, and has been used by pharma companies in early phase development for some years now. It establishes clear correlates of protection for vaccines and antivirals, can be used to inform go/no go decisions, and facilitates the up-or-down selection of study arms. It is now being used increasingly in asthma trials, based on the insight that asthma is an inflammatory disease in which viral diseases can cause exacerbations. These trials require a dedicated isolation suite, ideally located within a clinical pharmacology unit run by an experienced team.
The problems are illustrated by a Phase I randomized, double-blind, placebo-controlled trial that was carried out, first in 12 healthy normal volunteers, and then, in the second part of the trial, in 60 asthmatic subjects, on a monoclonal antibody targeting human TLR-3 for the prevention of asthma exacerbations. Subjects were given intravenous doses of the antibody ahead of inoculation with human rhinovirus type 16. The primary endpoint was safety and tolerability in part 1 of the trial and, in the second part, efficacy, as measured by pulmonary function testing and patient-reported outcomes. A range of secondary endpoints included pharmacokinetics, pharmacodynamics assessed via additional pulmonary function tests, the cold symptom assessment score, and fractional excretion of nitric oxide (a parameter for inflammation), biomarkers in nasal lavage, immunogenicity, and pharmacogenomics.
While the first part of the trial was successfully executed in 4 months, there were major hurdles, such as gaining regulatory approval to test a drug with a new mechanism of action combined with the viral challenge. There was, however, a major recruitment challenge, as 160 healthy consenting volunteers had to be tested to enroll just 12 individuals. This was mainly a result of a higher-than-expected positive antibody status. In the second part, the antibody status, when combined with further challenging inclusion and exclusion criteria, led to a 100% failure rate for recruitment after the assessment of 80 asthmatic patients. Thus, the second part of the trial could not be completed, highlighting the challenges associated with patient recruitment for certain viral challenge studies.
MEASURING LUNG DISPOSITION OF INHALED DRUGS
Sputum induction can be used to assess the concentration of inhaled drugs and outcome biomarkers in the lower airways.4 These biomarkers are inflammatory cytokines, and the induction technique is relatively simple: increasing concentrations of saline are inhaled. The simplicity and non-invasive nature of the technique have led to its widespread use in drug development. However, its reproducibility has been questioned for some time, and the preparation of sputum samples for bioanalysis is elaborate, time-consuming, and highly demanding, even for trained technicians. Few methodological studies have examined the influence of technical factors on the repeatability of sputum induction and collection, and thus there is no “gold standard” procedure.
However, good results are possible, as exemplified by one study carried out by SGS. A success rate of 29% was achieved in a patient group of 175, compared with just 10% in the literature, in non-smoking healthy volunteers, and 74% of 35 asthma patients, compared with 70% in the literature.5 A high success rate was also achieved in healthy volunteers who smoke, albeit with too few subjects to allow firm conclusions to be made. With care, the technique can be operated reliably.
A second methodology, local bronchial pharmacokinetics, can be used to determine the time-concentration profile of drugs and cytokines in bronchoalveolar lavage fluid (BALF) when predicting therapeutic efficacy. It also allows simultaneous assessment of the local and systemic pharmacokinetics of single and repeated doses of inhaled drugs. To execute this invasive technique, a pulmonologist with experience in bronchoscopy is required, as the scope has to be wedged in different positions in the bronchial tree in order to infuse saline distant from the bronchoscope and collect BALF samples.
As an example, a Phase I, single-center, open-label trial was carried out in male healthy volunteers in the SGS Phase I clinical unit, with the aim of evaluating the local and systemic pharmacokinetics of a nanobody targeting respiratory syncytial virus. The drug was administered to 41 healthy male volunteers as an oral inhalation of single or multiple doses, or as an intravenous infusion of a single dose. The aim was to determine local and systemic pharmacokinetics, as assessed by BALF and blood measurements, after single and repeated administration, as well as urinary pharmacokinetics, safety, and immunogenicity. In all, 44 healthy volunteers were included after 74 screen failures; three dropped out. The BALF procedure caused four moderate adverse events, three of which were fever and the fourth dyspnoea, and all recovered completely after a short time. The study was completed successfully, and delivered the robust data required for the PK/PD modelling of the study drug.
An alternative technique, functional respiratory imaging, relies on 3D segmented computer models of human organs. These are generated by combining different imaging techniques, including high-resolution computed tomography, MRI, and ultrasound, some of which include advanced computational tools developed in the aerospace industry, computational fluid dynamics, and finite element analysis.
This technique is currently able to support early phase clinical trials in asthma, COPD, idiopathic pulmonary fibrosis, cystic fibrosis, and sleep disorders. After constructing the models, airway resistance in different types of airways is measured, along with changes in lobar hyperinflation and lobar perfusion, with the further potential to measure local drug disposition. As it is non-invasive, it also has the potential to be used in later phase trials. When combined with positron emission tomography and inhaled radiolabelled drugs, there is further potential for the determination of pharmacodynamics.
SUMMARY
It is clear that classical primary respiratory endpoints are far from successful in exploratory and confirmatory studies. The new techniques that are being developed thus far have most potential in early phase, exploratory, clinical trials, but there may be the opportunity to apply at least some of them in the later stages of development. Only by the creative use of novel techniques to assist in the running of clinical trials will the major unmet medical needs be addressed in a timely and effective way.
REFERENCES
1. Jiemin Ma J, Ward EM, Siegel RL, Jemal A. Temporal trends in mortality in the United States. 1969-2013. JAMA 2015; 314:1731-1739.
2. Cazzola M, MacNee W, Martinez FJ, Rabe KF, et al. Society AT, COPD ERSTFooo. Outcomes for COPD pharmacological trials: from lung function to biomarkers. Eur Respir J. 2008;31:416-469.
3. Bergmann KC, Sehlinger T, Boelke G, Suberbier T. Clinical validation of a mobile environmental allergen exposure chamber. Allergy. 2015;70 (issue S101):540.
4. Chanez P, Holz O, Ind PW, Djukanovic R, et al. Sputum induction. Eur Respir J. 2002;20(Suppl 37):3s–8s.
5. Vos W, De Backer J, Poli G, Devolder A, et al. Novel functional imaging of changes in small airways of patients treated with extrafine beclomethasone/formoterol. Respiration. 2013;86:393-401.
To view this issue and all back issues online, please visit www.drug-dev.com.
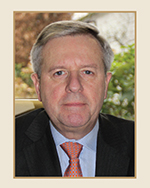
Dr. Robert Lins is Project Director, Respiratory Diseases at SGS Clinical Research. He is an MD and certified specialist in Internal Medicine, Nephrology, and Hypertension. He earned his PhD in Medical Sciences from the University of Antwerp, and is also a Fellow of the Belgian College of Pharmaceutical Physicians. Dr. Lins began his career over 35 years ago, working in Animal Clinical Pharmacology at the Heymans Institute in Gent, Belgium, and has since been continuously active in the field of Human Clinical Pharmacology. He first studied renal patients and later founded the SGS Clinical Pharmacology Unit in Antwerp, Belgium, where many innovative human pharmacology models were developed for the study of cardiovascular, metabolic, CNS, infectious, and most recently respiratory drugs. These include bronchial challenge methods, a local bronchial PK model, and sputum induction. Dr. Lins has published many articles about clinical pharmacology in these different areas of research.
Total Page Views: 2786