Issue:October 2024
PRECLINICAL/CLINICAL STUDIES - Preclinical Toxicology vs Clinical: Key Considerations in Using Lipid-Based Formulations
INTRODUCTION
Lipid-based formulations (LBFs) could improve the oral bioavailability of some molecules; however, a poor understanding of the technology and preconceived notions about its complexity have limited its use. To overcome those barriers, developers should first understand the developability challenges for their molecule, including the use of PBPK modeling to assist in identifying factors limiting bioavailability. Then a toolkit of enabling technologies, including LBFs, can be identified to overcome those factors and individually leveraged to create fit for purpose formulations for use in preclinical and clinical studies.
IMPROVING DRUG ABSORPTION FROM SELF-NANOEMULSIFYING DRUG DELIVERY SYSTEMS (SNEDDS) IN PRECLINICAL STUDIES
When creating a lipid-based formulation, self-nanoemulsifying drug delivery systems (SNEDDS) have repeatedly shown increases in exposure in both animals and humans. The isotropic mixture, loaded into a gelatin capsule or dosed directly from the preconcentrate, forms an emulsion droplet upon ingestion and entry into the gastrointestinal (GI) tract. The droplet is composed of the excipients with the hydrophilic surfactant on the surface. The aim is usually to have the drug remain inside the solution in the lipid emulsion particles, so it remains in a conducive environment that enhances the drug’s solubility and facilitates its dissolution and subsequent absorption.
In developing SNEDDS, solubility screening can determine which excipients to choose. One common problem is the solubility of the drug is not high enough. This can be a drawback if there are high amounts of excipients that need to be dosed to achieve the desired exposure or dose.
To address the problem of drug solubility in SNEDDS, the University of Copenhagen, Denmark, has been examining different ways to increase the load of the active pharmaceutical ingredients (APIs) at a low-dosing volume through methods including:
- Conventional SNEDDS, which contain about 80% of the drug in solution.
- A superSNEDDS suspension, which contains the same amount of a lipid but with a mixture of drug in solution and drug in suspension. For a toxicity study that does not require long-term stability, a superSNEDDS can be a good approach.
- A supersaturated SNEDDS, for which a higher drug load is achieved or there is more drug in solution than the equilibrium solubility. Applying the supersaturation principle can render more than two times higher a drug load in the SNEDDS, which makes them more relevant for preclinical exposure or toxicity studies.
- The chasing principle, which involves dosing an aqueous suspension of the drug and then chasing it with a lipid vehicle. This generates an environment in the gastrointestinal tract for increased dissolution or solubilization of the drug and, therefore, increased absorption.
Beyond superSNEDDS, there are additional ways to increase drug load. One way is by adding an amorphous solid dispersion (ASD) to a superSNEDDS. By adding an ASD into a superSNEDDS, one can combine the benefits of both systems, resulting in the amorphous drug with better stability, while the superSNEDDS ensures optimal delivery and a higher absorption in the gastrointestinal tract. When the combined ASD-superSNEDDS system was compared with conventional SNEDDS, the latter was found to have lower absorption rates.
Another method explored involves the use of a phospholipid (PL) complex, particularly suited for drugs with low lipid solubility. By creating a complex with PL, a greater amount of the drug can be loaded into the SNEDDS, enhancing its applicability for toxicity studies. Evaluating these techniques reveals distinct advantages associated with each, as illustrated in Figure 1.
LIPID-BASED FORMULATIONS: KEY OBJECTIVES IN PRECLINICAL TOXICITY STUDIES
It is important to understand that the doses set in toxicology studies are different from those in pharmacology studies. Pharmacology studies are done at pharmacologically relevant dose levels and are intended to demonstrate efficacious responses in animal models of disease. Toxicology studies, on the other hand, are conducted at toxicologically relevant dose levels and aim to characterize target-organ toxicity. This allows potential organ toxicity hazards to be identified in animals, and a human risk assessment with appropriate risk management strategies can be developed to ensure the safety of humans in clinical trials.
In toxicology studies, the dosage given to animals is of critical importance. The “high dose level” refers to the highest amount of a drug administered to animals during the toxicology study. This dose is particularly significant because it provides insights into the maximum adverse effects a drug can have, which is crucial for understanding its overall safety profile. When it comes to selecting a high dose level and justifying it to regulatory authorities, there are two International Conference on Harmonization (ICH) guidance documents available to consult — one for small molecule drug development, ICH M3(R2), and one for biopharmaceutical drug development, ICH S6(R1).1,2
ICH M3(R2) provides maximum recommended starting dose recommendations for first-in-human clinical trials of small molecule drugs based on preclinical data, primarily focusing on safety concerns. The rationale for this guideline is to recommend international standards and promote harmonization between nonclinical safety studies that support human clinical trials.1
Similar to ICH M3(R2), ICH S6(R1) provides dose recommendations for first-in-human clinical trials of novel drugs. However, the latter guideline is specific to biopharmaceutical drugs, which are more complex in nature and are associated with more unique considerations, including:2
- Selection of relevant animal species
- Age and physiological state
- Manner of delivery, including dose, route of administration, and treatment regimen
- Stability of the test material under the conditions of use
In general, for small molecules, the high dose level in a toxicology study can be set as either a maximum tolerated dose (MTD), maximum feasible dose (MFD), dose at which exposure saturation occurs, or a limit dose of 1000 mg/kg per day. For biologics, the rules for high dose justification are different due to the varying nature of the molecules. Margins can be set relative to efficacious doses and exposures in humans, in addition to other approaches for these types of agents.
When conducting a small molecule toxicology study, the high dose level should be an MTD, at least in the initial studies that will support a first-in-human clinical trial. MTD is the highest dose of a drug or treatment that does not cause unacceptable side effects.
Fit-for-purpose formulations in toxicology studies also vary from those in clinical formulations. Ideally, a robust toxicity program will provide for exposure margins at levels relative to therapeutic exposure. Higher margins create higher confidence and safety, so the goal in a toxicity program is to get the margin as large as possible.
For compounds with solubility and permeability limitations, unique toxicology formulations are sometimes required. These formulations are often different from those used in pharmacology studies and clinical studies. The goal of any toxicology formulation is to enhance bioavailability and increase the maximum feasible dose given to animals to maximize systemic exposure of the drug in the animal model. This is important as humans may be more sensitive to certain toxicities than animals are; therefore it is crucial to ensure that the animal models are exposed to the highest possible levels of the compound. By doing so, the safety margins that account for the differences in sensitivity between humans and animals can be established, ensuring a more comprehensive evaluation for human use.
Sometimes, the considered solubility and permeability limitations are not relevant to the doses and exposures to be evaluated in humans. In these cases, clinical formulations may contain fewer or even completely different excipients than the toxicology formulations. This is not uncommon and is completely acceptable from a regulatory perspective.
When deciding which lipids or other excipients to use, there are no regulatory constraints regarding what can be administered to animals in toxicology studies. Formulations can be as simple or as complex as they need to be to achieve the goal. To determine which excipients are acceptable for studies, it is helpful to consult historical control data and literature-based tolerability data. This involves comparing individual excipients and co-formulated excipients, the route and duration of administration, and the species.
Regulatory expectations demand that all inactive ingredients, including lipids, used in clinical formulations must be safe for human use. Therefore, “precedented” lipids and excipients should be chosen as opposed to novel ones that would require extensive safety evaluations. Every excipient and inactive ingredient considered should have been reviewed previously by regulators and requires approval for use in a commercial drug product. The US FDA maintains a publicly available inactive ingredient database (Figure 2). Users can refer to the database and search a particular excipient by name to clarify whether it has been approved by the agency.3
If using novel excipients is unavoidable, the FDA offers a pharmacology/toxicology guidance document that relates to nonclinical studies for the safety evaluation of pharmaceutical excipients.4
When transitioning from nonclinical to clinical studies, differences in formulation delivery technologies can arise. In toxicology studies for which an LBF is being used, it is important to discriminate between vehicle or formulation-based effects compared to its drug-related effects. Drug effects could be based on engaging the intended target (effects associated with the mechanism of action) or chemical-based effects. A robust control group that reflects the vehicle or the formulation only is required to discriminate between vehicle- or excipient-related effects in animals and effects that are ascribed to the active ingredient of the formulation. If nonclinical and clinical formulations differ, some of the findings that are associated with the vehicle or excipients in animals may not be relevant to human risk.
In GLP compliance toxicology studies conducted to support human risk, the reversibility of effects observed during the dosing phase should be evaluated. Assessments should include the reversibility of formulation-related and drug-related effects, although formulation-related effects may not be clinically relevant.
Some excipients are pharmacokinetically active in target organ profile assessments. They may not contribute to the pharmacology of the molecule or the toxicology of the formulation, but they can have effects that are related to the absorption distribution, metabolism, or elimination of the compound. Some LBFs can reduce the toxicity of certain drugs by changing the biodistribution of the drug away from sensitive organs. Additionally, some excipients have been shown to affect drug transporters and/or drug metabolizing enzymes. This should be considered when interpreting toxicology data and developing a human risk assessment.
If dosing toxicology studies to MFDs or to doses at which exposure saturation occurs, it is crucial to exercise due diligence during the development and selection of the toxicology formulation. To ensure regulatory compliance, it is generally recommended to conduct three different formulation efforts and include the resulting data in the regulatory submission. The ICH’s M3(R2) Q&A guidance document (Figure 3), explicitly states that regulators across the US, EU, and Japan expect such due diligence with formulation efforts.5
STRATEGIES TO LEVERAGE LBFS & MODELING TOOLS IN EARLY DEVELOPMENT
In the initial stages of drug development, it is critical to recognize LBFs as an integral part of the formulation toolkit. By utilizing modelling tools and developability assessment, formulators can swiftly identify the right formulation approach based on the requirements of the study and develop the transition roadmap from preclinical to clinical for a faster entry into first in human trials. Understanding what is involved in early stages of drug development empowers decision-making based on data and increases the likelihood of achieving favorable outcomes (Figure 4).
The first step in a drug development program is performing a developability assessment to help determine if a molecule is a candidate for drug development. To this end, physicochemical and DMPK characterization techniques should be synergistically combined with physiologically based pharmacokinetic (PBPK) modelling to predict how the molecule’s properties will influence its pharmacokinetic (PK) profile in vivo. If enabling technology is needed to enhance oral bioavailability, a toolkit of techniques are identified that can assist in overcoming the drug’s bioavailability limitations. While several technologies may be found suitable for the molecule, the intent of the in vivo study, either preclinical or clinical, will also play a role in guiding the technology selected for the study.
Key to a molecule developability assessment is to define the problem statement before formulation begins. Typical physicochemical parameters assessed in pre-formulation studies — such as biorelevant solubility, log P, pKa, and dissolution kinetics — are important attributes to a formulator. In addition, a review of drug metabolism and pharmacokinetics (DMPK) characteristics is important, because a drug’s bioavailability is not just about solubility; there are other factors that come into play, such as permeability, efflux, and metabolism.
After gathering physicochemical characterization data, DMPK characterization data, and PK profiles in animal species, PBPK modelling can be used to predict potential exposure in humans and helps formulators identify the key parameters influencing bioavailability.
Beyond candidate selection, PBPK modelling provides a rational basis for target formulation development, looking at areas including absorption, PK profile, and formulation impact.
When formulating a drug for oral administration into animals and humans, it is important to understand the differences between species. Variations in size and physiology, such as differences in enzyme expression and activity, must be taken into account when designing the study. This is particularly important when utilizing a LBF that relies on the digestive process to enhance drug solubility. To address these physiological variations, as well as achieve dosing levels appropriate for the specific study, formulators could greatly benefit from having access to a diverse range of formulation technologies.
A thoughtful approach is required to realize the best preclinical outcomes when using lipidic systems (Figure 5) in animal studies.6-8 To overcome physiology issues, PBPK modelling, tailored in vitro lipolysis tools, use of relevant species, and a reduction of the dose volume, especially in smaller species, can be used. To address solubility issues, screening lipid formulations at higher concentrations, lipid suspension, and co-dosing an ASD with an LBF can be effective.
In bridging the gap between preclinical and clinical formulations, it is advisable to revisit the enabling toolkit to ensure the drug product presentation for clinical is not overcomplicated based on the needs of the high dose GLP toxicity studies. Toxicology studies may require more aggressive enabling formulations to achieve exposure but often the clinical formulation approach can be simplified.
Supporting scientific justification will be important to bridge the gap between preclinical and clinical studies. For example, PK data obtained from a different enhancing technology may be used to estimate human exposure with LBF through PBPK modelling. The effect of any functional excipients introduced into the clinical drug product and the anticipated impact on safety data should also be considered. Additionally, it is recommended to use approved lipidic excipients with a regulatory precedent when feasible. If using an alternative technology to enable high dose GLP studies, generating lipid excipient compatibility stability data while GLP is ongoing can help streamline clinical formulation activities. This will also assist with identifying potential excipient interference peaks in analytical methods early on.
This figure demonstrates a typical enhancing technology toolkit for clinical formulations (Figure 6), including LBF, spray dried dispersion (SDD), hot-melt extrusion (HME), and particle size reduction (PSR). It is important to choose the right scientific solution to match the problem. Other attributes, such as the amount of API, time, and costs, also factor into the strategy for the clinic.
LBFs also offer a unique advantage of enabling a rapid path to the clinic owing to their liquid form. This liquid solution can be conveniently prepared on-site at the clinical facility by filling into air-filled gelatin capsules. This approach not only minimized the amount of API needed, but also significantly cuts down the time required for clinical preparation.
SUMMARY
One of the most promising technologies for enhancing the oral bioavailability of molecules is the use of LBFs. They work by increasing the solubility of the drug in the gastrointestinal tract, which makes it easier for the drug to be absorbed into the bloodstream, thereby improving its therapeutic efficacy. When developing LBFs, it is essential to consider the specific requirements of preclinical toxicology and clinical studies. Preclinical studies usually involve higher doses of the drug than clinical studies, and as such, the solubility and permeability limitations that may be relevant to the preclinical studies may not be as significant for the doses and exposures to be evaluated in humans. This implies that the clinical LBF formulation may contain fewer or even completely different excipients than the toxicology formulations.
Moreover, the use of modelling tools can be immensely helpful in designing LBF formulations that are more likely to be successful. These tools can predict the drug’s absorption in the gastrointestinal tract and provide insights into the key sensitivity parameters that influence absorptions. By keeping all these factors in mind, developers can leverage the potential of LBFs to improve the oral bioavailability of molecules and accelerate the delivery of drugs to patients in the early stages of development.
REFERENCES
- International Conference on Harmonisation of Technical Requirements for Registration of Pharmaceuticals for Human Use. Guidance on nonclinical safety studies for the conduct of human clinical trials and marketing authorization for pharmaceuticals M3(R2). Published Jun 11, 2009. Accessed online August 28, 2023. https://database.ich.org/sites/default/files/M3_R2__Guideline.pdf.
- International Conference on Harmonisation of Technical Requirements for Registration of Pharmaceuticals for Human Use. Preclinical safety evaluation of biotechnology-derived pharmaceuticals S6(R1). https://database.ich.org/sites/default/files/S6_R1_Guideline_0.pdf.
- FDA. Inactive ingredient search for approved drug products. Last updated July 21, 2023. Accessed August 28, 2023. https://www.accessdata.fda.gov/scripts/cder/iig/index.cfm.
- FDA. Nonclinical studies for the safety evaluation of pharmaceutical excipients guidance document. Published May 2005. Accessed online August 28, 2023. https://www.fda.gov/regulatory-information/search-fda-guidance-documents/nonclinical-studies-safety-evaluation-pharmaceutical-excipients.
- International Conference on Harmonisation of Technical Requirements for Registration of Pharmaceuticals for Human Use. M3(R2) Implementation Working Group M3(R2) guideline: guidance on nonclinical safety studies for the conduct of human clinical trials and marketing authorization for pharmaceuticals questions & answers (R2). March 5, 2012. Accessed online August 28, 2023. https://database.ich.org/sites/default/files/M3_R2_Q%26As_R2_Q%26As_0.pdf.
- Nanjwade BK, et al. Functions of lipids for enhancement of oral bioavailability of poorly water-soluble drugs. Sci Pharm. 2011;79(4):705-727.
- Chen XQ, et al. Application of lipid-based formulations in drug discovery. J Med Chem. 2012;55(18):7945-7956.
- Jannin V. Complex Interplay Between Solubilization, Digestion, Supersaturation and Absorption of Poorly Soluble Drugs with Lipid-Based Formulations. Curr Drug Deliv. 2018;15(6):749-751.
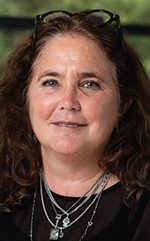
Dr. Anette Müllertz is professor in oral drug delivery and industrial relations at the University of Copenhagen, Denmark (UCPH) and head of Bioneer:FARMA, a business unit of Bioneer A/S, which is a research-based, non-for-profit service provider within the area of biomedicine and pharmaceutical development. She is heading the Physiological Pharmaceutics Research Group at UCPH, focusing on developing oral lipid-based drug delivery systems and predictive biopharmaceutics tools. She has >250 publications in international, peer-reviewed journals (h-index: 67, 13815 citations, (Google Scholar 9/5-23). She is/has been supervising 12 post docs, 53 PhD students and numerous master students, primarily at the University of Copenhagen, but also at other universities. She is/has been involved in multiple national and international research consortia, eg, the EU sponsored Innovative Medicines Initiative Consortium Oral Biopharmaceutics Tools and Horizon 2020 MSCA training network COLOTAN.
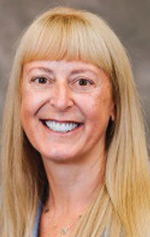
Dr. Grace Furman is a Fellow at the American Association of Pharmaceutical Scientists and recipient of the AAPS Lipid Based Drug Delivery Award. She has been involved in the transition of two AAPS Focus Groups into Communities; Lipid-based Drug Delivery and QbD and Product Performance. She is Editor of the Journal of Drug Delivery Science and Technology (IF2023: 5.062) and earned her PhD in Biomedical Sciences with a specialization in toxicology from Northeastern University. She also holds a BS in Toxicology and has been a Diplomate of the American Board of Toxicology since 2000. She is an active member of the American College of Toxicology (ACT) and has served ACT as a 2-term Council member. She is also an active member of the Society of Toxicology and the Southern California Chapter of the Society of Toxicology and has held several Executive Council positions. She is Chair Emeritus of the San Diego Biotechnology Discussion Group and a founding member of San Diego’s Women in Biosciences. She designed and instructed the Toxicology classroom and distance-learning courses offered through University of California/San Diego Extension’s Drug Discovery and Development and AMDET Process Certificate Programs.
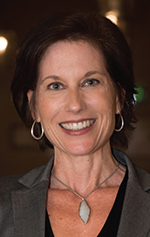
Lisa Caralli is Science and Technology Director, Pharmaceutics at Catalent San Diego. She has over 30 years of industry experience in pharmaceutical research and development. Her area of expertise is analytical methods and formulation development of small molecules and peptides. In her role, she works with pharmaceutical companies to identify the right development pathway for their early development drug candidates and advances new technical offerings within Catalent.
Total Page Views: 1812