Issue:March 2024
PREFILLED SYRINGES - Making a Prefilled Syringe Smart: Technological Solutions to Advance Patient Care & Clinical Trial Outcomes
INTRODUCTION
A prefilled syringe (PFS) is widely employed in healthcare. With the raising prevalence of injectable biological products, the PFS is becoming the primary mode of drug administration. The global PFS market is projected to reach $13.33 billion by 2028, with a compound annual growth rate (CAGR) of 11.11%.1 PFS is growing in preference over conventional needle and vial delivery systems because it preserves drug sterility, limits drug waste due to overfilling, improves needlestick safety, simplifies the administration process, and reduces the likelihood of dosing errors. A PFS has particularly found two major fields of application: clinical trials and chronic disease therapy. Adherence to the dosing regimen, as well as compliance to the injection protocol, are essential for treatment success in both these use-cases. Hence, the request for a connected, smart PFS is growing just as much to tackle poor medication adherence and compliance issues.
MARKET NEEDS: CLINICAL TRIALS & CHRONIC DISEASE MANAGEMENT
In a clinical trial, one of the major hurdles for pharma companies is the lack of objective data on treatment adherence based on real measurements rather than informal patient feedback. The latter represents the only thing pharma companies can rely upon still to date. Non-adherence to the dosing regimen can largely affect the assessment of drug efficacy.2 When study participants do not perform injections as prescribed, this can result in underestimated drug efficacy, which compromises the outcome of the clinical trial and ultimately delays the drug time-to-market.
In chronic disease management, adherence and compliance errors are equally frequent. A patient is usually required to perform self-injection weekly, without receiving assistance from a healthcare professional (HCP). This is when a connected PFS can come to the rescue: it allows to automatically keep record of injections by possibly fostering patient engagement.
A connected PFS provides a reliable means to solve the challenge of monitoring treatment compliance and adherence in both clinical trials and home-based therapies. Nonetheless, there is still a technology gap to fill in the drug delivery industry. The market is certainly populated with connected pens and autoinjectors, but where the industry is yet lacking is in the integration of sensors and connectivity features on a PFS. In recent years, Flex has investigated several variants of a smart PFS implementation to address different market requirements and usability scenarios. The following provides a review of technological proposals for a smart PFS reference design platform, including the premises and the technical aspects associated to the implementation of it.
DESIGN & DEVELOPMENT STRATEGY ON SMART PFS
This section reports the strategy, the rationales, and the conclusions that led the Flex team to investigate multiple smart PFS variants, based upon different connectivity and architectural options. Major criteria that were set to guide the design and development study were:
- The addition of digital features shall not require a modification of the PFS
- The addition of digital features shall have no (or least) impact on the overall form-factor
Based on this, two concept ideas have been explored for the implementation of a smart PFS, mostly relating to the section of the PFS delivery system where the digital features should be fitted in (Figure 1)
- Separate electronic module to mount on the flange of the plunger rod as add-on
- Custom plunger rod which incorporates electronics features
Other meaningful criteria that were assessed to narrow the concepts down to those finally selected and fully developed were:
- Quantity of info: it describes the added value that the technological solution is providing in terms of data that can be tracked.
- User experience: it describes the impact the added technology is having on the usability of the PFS, and the overall injection experience.
- Ease of implementation: it describes the degree of complexity of the technological solution in terms of expected engineering effort, and estimated time-to-market.
- Cost: it describes the additional cost of technology being added to the PFS.
The Flex team brainstormed a shortlisted set of info to collect from the PFS, selecting those that appeared to be most relevant to the chronic disease management and/or clinical trial applications. The choice leaned toward:
- Drug product info (digitally encoded into the syringe)
- Start and end of injection
- Force applied to extrude the drug
Volume of injected drug
On the connectivity end, it was decided to investigate both Near-Field Communication (NFC) and Bluetooth Low Energy (BLE) based devices to address two different types of market:
- NFC as a very affordable, more eco-friendly (due to the absence of batteries) and easier to implement solution, which however requires a voluntarily action on the user side (ie, tap) in order to transfer data from the PFS to an NFC-enabled smartphone.
- BLE as a more premium solution that offers a smoother user experience, and more sensing capabilities, with the downside of a higher price-point and the need of integrating batteries (which in principle results in a higher environmental impact).
SMART PFS IMPLEMENTATION: A) ADD-ON MODULE VS. B) CUSTOM PLUNGER
Add-On Module
One possible device embodiment is an electronic module to install as add-on to the PFS’s plunger rod. The add-on can be assembled immediately after the drug filling. For the sensor part, in its simplest implementation, the device can enable the sensing of the injection completion. For the connectivity part, two alternative concepts for the add-on supporting either NFC or BLE connectivity are possible.
The NFC version can incorporate a printed NFC inlay, with an NFC tag connecting to a printed sensor switch for detecting the end-of-injection event. The NFC add-on does not incorporate any battery, with the tag chipset that gets powered by the NFC field of the reader (eg, NFC-enabled smartphone) as soon as it comes in close proximity with the PFS. The user can tap the PFS
with the smartphone before executing the injection to verify the authenticity of the drug (ie, product info), and after the injection to log the end-of-injection event together with a timestamp.
The BLE version allows for more sophisticated sensor implementations. The sensing of the injection completion can be accomplished in multiple ways, either through mechanical, magnetic, or capacitive technologies. To streamline cost and engineering simplicity, the add-on could implement a miniaturized switch, which activates when the plunger reaches the stopping point. The embedded software is then able to associate this trigger event to the indication that the injection is completed. In contrast to NFC, the BLE add-on incorporates a coin-cell battery, and connects to a BLE mobile app for data transmission.
A more advanced implementation of the BLE add-on could feature a miniaturized force sensor to measure the drug delivery force applied throughout the injection (up until the stopping point). This is becoming particularly relevant considering new therapies are often relying upon injections of highly viscous biological formulations. Force measurements can be accomplished with a variable capacitor sensor. The add-on discussed herein is based on a sensor principle that leverages a spring-activated sheet-metal plate facing the battery holder to form a parallel-plate capacitor subsystem as shown in Figure 2.
The whole sensing structure is extremely small. The use of a battery holder as second electrode reduces the parts count, expected to lower the cost of the bill of material, and the assembly time.
Custom Plunger Rod
Another possible smart PFS embodiment is a custom-made plunger rod, which incorporates electronics features. The connected plunger is meant to replace a purely mechanical plunger rod: compatibility with commercially available PFS must be guaranteed. Two alternative concepts based on either NFC or BLE are possible also in this case. Regardless of the connectivity type, the smart plunger rod discussed herein was specifically designed to be fully compatible with 1-mL PFS, and it has the same length and diameter of existing plungers.
The NFC version could be implemented as simple as having an NFC tag encapsulated into the flange of the plunger rod. The tag’s memory could be programmed at factory level with product information, such as the drug’s name, the drug’s manufacturing or filling date, and the expiry date. Then, the information encoded into the smart plunger can be read as usual by an NFC-enabled smartphone. This is a passive NFC solution, with no sensing capabilities associated to it. Again, no battery is necessary for an NFC-based smart plunger.
The BLE version could be a more feature-rich solution, with the plunger that could integrate a printed circuit board (PCB) with sensors and BLE connectivity. The BLE-enabled plunger incorporates the PCB and a coin-cell battery into the inner volume of the rod as illustrated in Figure 3.
For the sensor part, the circuit board could implement a positional sensor to measure continuously the exact drug volume that has been delivered as the user presses the plunger rod. Besides the start and end of injection, the device can record even partial dosing (Figure 4), which aids to identify compliance errors.
COST ANALYSIS
The acceptance of smart features into a PFS has been limited so far by the relatively high cost that is required to incorporate such features. From the design and development standpoint, there is yet an effort to lower the system cost to overcome the market entry barrier for an electronically assisted PFS. For the electronic part, reducing the cost of connectivity is essential. With this push toward disposable devices, silicon vendors are delivering chipsets with a simplified silicon architecture, which translates in a very low price-point. This holds particularly true for BLE, which is usually a more expensive technology compared to NFC. A custom-designed PCB antenna is also a sound decision to further reduce the system cost. The design of a 2.4-GHz antenna on such small devices is not trivial. It is crucial to predict the field loading effect that is generated by all the elements surrounding the antenna, such as the battery, the plastic enclosure, as well as the human body (eg, user’s hand) acting as absorbers from the radio-frequency (RF) standpoint. All these elements will have a strong effect in de-tuning, and overall lowering the electromagnetic radiation of the antenna. The antenna can be designed with the aid of RF simulation tools, such as Ansys HFSS.3 This is a powerful tool that allows to build an accurate 3D digital model of the smart PFS to streamline the design of the antenna to achieve optimal radiation efficiency.
CONNECTIVITY TO CLOUD: DATA SECURITY
The smart PFS must be an integral part of an end-to-end digital ecosystem. This combines the device with software and cloud services that can ingest PFS’s data to elaborate meaningful insights about the therapy. Data collected by a smart PFS are sent to a companion mobile app (via NFC or BLE), with the smartphone that then bridges the data to a back-end cloud system over the Internet, as shown in Figure 5.
For the simple fact that the device is operating into the network, it becomes potentially exposed to cyber-attacks. It is mandatory to secure thoroughly the communication channel up to the cloud’s services that will use the data. However, adding security to a smart PFS presents some technical challenges. There is the need to implement advanced security protocols, activate data encryption, and store digital certificates securely. Careful consideration shall be paid to the selection of the silicon itself. It is important to rely on a chipset that embeds the appropriate security support, including encryption engine and secure storage of crypto keys. Regardless of the type of implementation (as described in previous sections), the smart PFS can implement industry-standard AES128-EAX scheme, which provides both mutual authentication with the cloud and data encryption to protect the transmitted data.
DESIGN FOR ENVIRONMENT
The benefit of using a smart PFS must not come at the expense of the environment. As a single-use device, a smart PFS tends to increase the amount of plastic and electronic waste. This mandates the implementation of design for environment (DfE) best practices to produce an environmentally friendly smart PFS. Starting from the material selection, one opportunity is to use environmentally sustainable resins generated from bio-based feedstock with a mass balance approach.4 This is totally equivalent to traditional plastic in terms of grade, quality, and mechanical performance. Although, it has a lower environmental impact because it is generated from biomass and waste cooking oils, in contrast to traditional plastic which is made of carbon-based sources. The device (whether it is shaped as add-on or custom plunger) needs also to be designed to be easily disassembled at the product end-of-life. For example, no welding or hard fixing is used to seal the plastic elements together. The device can rely upon snap-fit features to ease the opening of the housing, and ultimately the separation of the plastic from the electronics during disposal. The product might still have some residual value after use:
- Both the add-on and the custom plunger could be separated from the PFS, potentially opening the opportunity to apply it as re-usable item on a new PFS.
- The plastic might be recycled (e.g., chemically, mechanically) and reused as raw material in a non-medical market.
SUMMARY
PFS is becoming the norm for the administration of biological drug products via injections. Important factors driving the growth in the PFS market are the continuing prevalence of PFS-injectable drugs to treat chronic diseases, and the adoption in clinical trials. While these might be seen as different use cases, they share a common request: they demand accurate digital data to demonstrate medication compliance and adherence. A smart PFS provides HCPs with reliable data to monitor a patient’s in-take behaviors, and clinical study teams with all data they need to assess the efficacy of new investigational drugs and therapies. Adding digital features to small-volume PFS is not trivial. Smart PFS design must be approached within a multidisciplinary framework. Well-proven design and development expertise in this field becomes paramount to surf this unique market opportunity. Recognizing that a one-size-fits-all approach does not always work, Flex is proposing a variety of technological solutions for a connected, smart PFS that can be turned into end-products. Preparing now for the transition from regular to smart PFS is essential.
REFERENCES
- Prefilled Syringes Market Size & Share Analysis – Growth Trends & Forecasts (2023 – 2028), Mordor Intelligence, https://www.mordorintelligence.com/industry-reports/prefilled-syringes-market
- Eliasson, L., Clifford, S., Mulick, A., Jackson, C., & Vrijens, B. (2020). How the EMERGE guideline on medication adherence can improve the quality of clinical trials. British Journal of Clinical Pharmacology, 86(4), 687-697, https://bpspubs.onlinelibrary.wiley.com/doi/full/10.1111/bcp.14240.
- Denna E, “Using Simulation and Modelling Versus Prototyping for Medical Radio Frequency Design”. ONdrugDelivery, Issue 124 (Sep 2021), pp 67–71, https://www.ondrugdelivery.com/using-simulation-and-modelling-versus-prototyping-for-medical-radio-frequency-design/.
- “Enabling a circular economy for chemicals with the mass balance approach”, Ellen MacArthur Foundation, White Paper (2019), https://emf.thirdlight.com/link/f1phopemqs36-8xgjzx/@/preview/1?o.
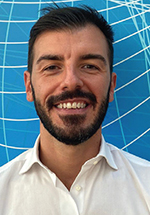
Salvatore Forte is Innovation Manager at Flex, leading an R&D engineering team in the company’s Design Center in Milan, which works to bridge the gap between technology breakthroughs and successful design implementation for medical devices. He has a solid technical background in analogue and digital electronics, specializing in embedded system design for power-sensitive medical devices, such as wearable health monitors, disposable point-of-care, and automated drug delivery. He earned his MS in Electrical Engineering from University of Naples Federico II (Italy).
Total Page Views: 7922