Issue:April 2023
DRUG-ELUTING IMPLANTS - Delivery of RNAi Therapeutics Through Drug-Eluting Implants
INTRODUCTION
The past few years have seen a rapid uptick in focus on RNA therapeutics stemming from the success of the mRNA COVID vaccine. As a result, additional RNA drug classes are being investigated to treat a wide range of conditions using a different modality. While mRNA therapies are only now beginning to emerge commercially outside of infectious diseases, the first oligonucleotide-based RNAi therapeutics were approved in 1998 (antisense oligonucleotide) and 2004 (aptamer). There are now 13 FDA or EMA approved oligonucleotide drugs spanning siRNA, antisense oligonucleotides (ASOs), and aptamers.1 Additionally, miRNA mimics and inhibitors are an emerging class of yet-to-be-commercialized therapeutics with multiple drugs in clinical development. However, ASOs and siRNA continue to represent the largest share of oligonucleotide drugs.
The new wave of next-generation oligonucleotide therapeutics has spurred market growth and is projected to result in a global market size of $7.2 billion in 2026.1 Advances in oncology, rare diseases (primarily in central nervous system, cardiovascular, and metabolic conditions), and ophthalmology are drivers of this growth.2,3 Additionally, the growing focus on personalized medicine and the need to target specific patient sequences and undruggable targets can be addressed by oligonucleotide therapeutics.
LIMITATIONS OF CONVENTIONAL DELIVERY METHODS
RNA that interferes with or modifies gene expression works through different mechanisms of action, yet all must avoid clearance by off-target organs and be delivered to the correct organ and tissue site. Each generation of RNAi therapeutics utilizes a variety of new backbone or nucleotide chemical modifications leading to improved therapeutic efficacy, specificity, and stability, yet challenges still remain in reducing off-target toxicities and avoiding broad distribution of therapeutics to multiple organs. These issues have resulted in inflammatory side effects, such as thrombocytopenia and glomerulonephritis, due to drug circulation and accumulation in non-target organs. Additionally, broad distribution of ASOs requires high dosing to ensure the concentration of ASOs needed to produce a physiological, therapeutic effect actually reaches its intended site.
For example, the observed biodistribution from the systemic administration of single-stranded, phosphorothioate-modified ASOs is broad, with the highest ASO concentrations in liver and kidneys. To solve this, several companies have tried to create conjugated ligands that target receptors in the desired tissue. However, adequate receptors do not exist for all tissue types, and some receptors may also be expressed in non-target tissues. Physiological barriers and heterogeneous targets also add complexity to receptor-ligand-based approaches.
IMPLANTS FOR RNAi THERAPEUTIC DELIVERY
Current RNA therapeutic challenges can be overcome through drug delivery approaches. Localized therapeutic delivery of these therapies through an implant (Figure 1) provides an innovative route of administration for chronic conditions that are difficult to dose adequately. Implants used to facilitate intrathecal or intratumoral delivery can bypass physiological barriers that typically reduce drug efficacy. This would allow for a higher concentration of the therapeutic at the local site of interest and also reduce the risk of toxicities. An implant also provides localized, sustained release of the RNAi that may potentially reduce the amount of drug that needs to be delivered as more of the drug reaches the target site resulting in improved efficacy.
Localized delivery via an implant can improve ligand-conjugated and non-conjugated RNAi therapeutics. For ligand-conjugated therapeutics, a localized approach situates the implant directly at the organ site of interest. This proximity may allow for lower dosing requirements as a higher fraction of the drug will reach the cells of interest than in a systemic approach. For non-ligand conjugated RNAi therapeutics, situation of the implant at the site of interest will also allow for less accumulation of the drug in organs not indicated for treatment. Regardless of conjugation approaches, localization may result in reduced toxicity and a lower immunogenicity profile.
USE CASES
Rare Diseases
RNA therapeutics are well established in the rare disease market, with drugs like Spinraza (Ionis & Biogen), Onpattro (Alnylam), and Vyondys 53 (Sarepta Therapeutics). The majority of RNA therapeutics in the pipeline have been granted FDA orphan designations.3 These drugs utilize ASOs and siRNA that are typically modified to confer improved stability and cellular uptake. Sustained delivery of these products in rare diseases may further elongate the interval between treatments and mitigate off-target effects (eg, thrombocytopenia). For example, implant delivery of ASOs for some rare CNS diseases may reduce the dosing frequency needed via delivery through various routes of administration (eg, intrathecal). Implant delivery for rare musculoskeletal diseases may also improve efficacy of current treatments by providing continuous, low-dose release for improved muscle uptake.
Oncology
Similar to rare diseases, there are high hopes for RNA therapeutics in oncology. The volume of research advancing new oncology therapeutics based on RNA includes more than 75 ongoing clinical trials and more than 160 ongoing research/preclinical studies. Many different types of RNA have been shown to be dysregulated in tumors, therefore a wide range of potential mechanisms can be used to treat tumors. Areas under investigation include inhibiting the proliferation of tumor cells, preventing the metastasis of malignant tumor cells, inducing tumor cell apoptosis, reconstructing the tumor microenvironment, tumor cell reprogramming, expression disruption, and angiogenesis inhibition.4 In addition, combining RNA therapy with existing medicinal therapies has the possibility to decrease drug resistance over time.
One particular class that has presented a significant amount of clinical data to date is siRNA-based therapies targeting lung, breast, and pancreatic cancers. For example, an siRNA targeting PD-1 has been shown to increase anti-tumor activity of tumor infiltrating lymphocytes (TILs) against certain tumor cells.5,6 siRNA has also been used to knock down PD-L1 and CD47 on the cancer cell surface to boost the efficacy of anti-PD-L1 and anti-CD47 inhibitors.5,7 Localized delivery of these therapies via an intratumoral implant may further improve efficacy and reduce toxicities to other sites. Research and trials are ongoing to help better identify new molecular targets of RNAi therapies for the reduction of tumor size.
Ophthalmology
siRNA and ASOs have been investigated as potential therapeutic modalities for targeting posterior chronic eye conditions like geographic atrophy (GA) secondary to dry macular degeneration. Zimura, a pegylated RNA aptamer, has shown favorable Phase 3 clinical trial results and may transform the landscape of dry AMD and RNA in ocular indications if approved.8 The oligonucleotide ocular pipeline is also buoyed by Roche’s Ionis partnered drug for GA.9 Sustained oligonucleotide delivery from an implant has the potential to extend treatment duration beyond 6 months, which would reduce treatment burden for patients with chronic eye conditions.
FUTURE OUTLOOK
Beyond sustained delivery of oligonucleotides, implants have the potential to improve the delivery of mRNA therapeutics. Chronic conditions using mRNA for protein replacement could benefit from an implant depot approach, which would provide continuous delivery in a localized manner. This localized manner of delivery could also reduce the common immune response associated with bolus injections and mitigate the need for targeting ligands. Drug delivery approaches used to elute large molecules, like VitalDose® EVA for monoclonal antibodies, can also be used to elute other large molecules like mRNA. The future of RNA for disease treatment is vast and constantly growing. As research continues in this therapeutic space, the consideration of drug delivery options can be crucial to the efficacy of treatment. Localized delivery via a drug-eluting implant is only one option to be considered, but it holds a great deal of potential to help optimize RNA therapeutic success and must not be overlooked.
REFERENCES
- Oligonucleotides – Global Market to 2026. BCC Publishing Staff. September 2021.
- Roberts et al. Advances in Oligonucleotide drug delivery. Nature Reviews (2020).
- Wang F et al. RNA Therapeutics on the Rise. Nature Reviews (2020).
- Tian Z et al. Insight Into the Prospects for RNAi Therapy of Cancer, Frontiers in Pharmacology (2021); 12.
- Liang X et al. RNA-based pharma for tumors: From bench to clinic and back, Biomedicine & Pharmacotherapy (2020); 125.
- Ligtenberg M et al.Self-Delivery RNAi Targeting PD-1 Improves Tumor-Specific T Cell Functionality for Adoptive Cell Therapy of Malignant Melanoma, Molecular Therapy (2018); 26.
- Lian S et al. Simultaneous block of CD47 and PD-L1 increase innate and adaptive cancer immune responses and cytokine release, EbioMedicine (2019), 42.
- Jaffe G et al. C5 Inhibitor Avacincaptad Pegol for Geographic Atrophy Due to Age-Related Macular Degeneration. Ophthalmology (2021); 128 (4).
- Jaffe G et al. Development of IONIS-FB-LRx to Treat Geographic Atrophy Associated with AMD. IOVS (2020).
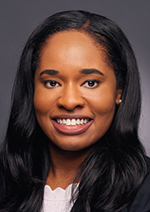
Dr. Cyonna Holmes earned her PhD in Biomedical Engineering from the University of Texas Southwestern Medical Center (Dallas, TX) and her BS in Bioengineering from Stanford University (Stanford, CA). At Celanese, she serves as Global Strategy Lead for Ophthalmology, Rare Diseases, and RNA Therapeutics. She has experience in the areas of drug delivery, lifecycle management strategy, new product development, and market entry strategy. She has published articles in peer-reviewed journals.
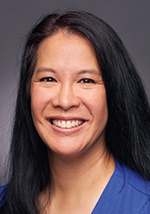
Karen Chen earned her MS in Formulation Science from Fairleigh Dickinson University (Teaneck, NJ) and her BS in Biology and Chemistry from Rutgers University (New Brunswick, NJ). At Celanese, she serves as Global Strategy Lead for Oncology. Her experience spans new product development as well as market entry and expansion strategies for functional excipients and drug delivery.
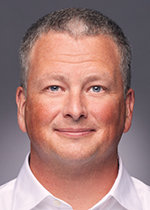
Dr. Brian Wilson earned his PhD in Biochemistry from the University of Notre Dame (South Bend, IN). He has 11 years of API experience in the areas of formulation development, drug delivery, and new product development. At Celanese, he serves as a New Business Development Partner for the pharma and medical businesses. He holds patents and has numerous published articles in peer-reviewed journals.
Total Page Views: 2266