Issue:October 2019
MODIFIED RELEASE - Alternative Strategies for Development of Modified-Release Dosage Forms
INTRODUCTION
Modified-release (MR) formulations are in high demand. For formulators, they enable drugs to be released in the optimal gastrointestinal (GI) locations to achieve and maintain desirable plasma concentrations for extended periods, avoiding undesirable excursions outside the therapeutic range. For patients, MR formulations provide the convenience of infrequent dosing with potentially greater efficacy and fewer side effects than similar, immediate-release delivery systems.
An impressive variety of MR formulations are possible, thanks to ongoing technological developments. Strategic selection of excipients and delivery technologies can yield MR formulations that fulfill very specific performance requirements, such as gastro-retention and sustained-, pulsatile-, or delayed-release formats.
Nonetheless, throughout the past 20 years, the fundamental methodology for developing these formulations has stagnated. Initial formulations continue to be founded upon in vitro and preclinical test results, despite evidence that these data correlate poorly with pharmacokinetic (PK) drug performance in humans.1 The following will discuss a range of available formulation technologies, the challenges in MR formulation development, and the use of a design-space approach with on-demand manufacturing. This methodology enables critical-to-performance formulation adjustments during clinical conduct, saving time and cost, and reducing risk in MR drug development.
THE CHALLENGES OF MR FORMULATION
An ever-increasing number of polymers and formulation technologies allow finely tuned control of many aspects of drug release. Selecting and/or combining these technologies offers great potential for optimized oral drug delivery. However, managing all the variables and interpreting in vitro, preclinical, and available human clinical data to define a formulation strategy capable of achieving the desired PK performance is more difficult than many developers expect. Accurate performance prediction is crucial because miscalculations in planning for development or manufacturing are costly and often cause delays.
Abundance of Technology: A Blessing & a Curse
Off-the-shelf and proprietary polymers help MR developers achieve their PK goals. Certain polymers are better suited for sustained or delayed release and may be designed to deliver APIs to specific GI target areas, depending on physicochemical, biomechanical, and human physiological factors influencing the site of release. The range of solid dosage forms offers further layers of complexity. This ever-expanding array of tools makes many MR modalities possible. Some options are shown in Table 1.
Each delivery format has its own idiosyncrasies. In the first instance, understanding the target PK profile is crucial. What plasma concentration time profile does the formulation need to deliver? Experience and expertise are then required to select and implement a rational formulation program based upon API characteristics, such as solubility, stability in stomach acid, particle size, and bioavailability. Furthermore, human physiology factors (such as an absorption window, drug transporters, and enzymes in the GI tract, and intestinal motility) can impact a product’s performance as it transitions through the intestine. Given these variables, being sure the formulation performs in vivo as it did in vitro can be challenging. A good CDMO will help developers find the best approach and select a technology to achieve the desired performance.
The Rocky Transition From Lab to Human
What is the best way to predict a formulation’s clinical performance? Ultimately, how an MR delivery system will perform in humans is unknown until clinical PK data becomes available, no matter what the in vitro and preclinical data indicated. Successful performance depends on an interplay between the drug molecule, the formulation, and the gastrointestinal environment. Despite their long-term, widespread use for this purpose, in vitro and preclinical studies cannot always be relied upon to predict how formulations will perform in humans.2,3
There are many reasons why animal studies are not necessarily predictive of how a drug will behave when administered to humans. The pHs in the various segments of animals’ GI tracts differ from those in the corresponding segments in humans. Animals’ stomachs exert different peristaltic wave forces and have different gastric-emptying rates as well, so diffusion rates, release rates, and PK profiles are not always representative. The best model for a human is a human.
A traditional clinical investigation for developing an MR system would be to test 2-3 fixed-formulation prototypes, such as a slow formulation, a fast formulation, and perhaps one in between based on in vitro and preclinical data, and hope one achieves the desired PK profile. But if these combinations miss the target PK profile, an additional time-consuming development cycle will be required, incurring a delay of 12-18 months and additional outsourcing costs of $1.5-$2.5 million. In contrast, using a design space concept will produce the required PK profile efficiently, in a single development cycle.
THE DESIGN SPACE STUDY MEETS REAL-TIME MANUFACTURING
The most obvious question when planning to develop an MR dosage form is: What is the starting composition and dose? In vitro dissolution studies are an invaluable tool to inform formulation development. However, there is considerable uncertainty as to whether a fixed formulation that achieves the desired release rate in vitro will also deliver the required performance in humans. If the starting formulation misses the mark completely, a second clinical study with an adjusted starting formulation will be required, leading to escalating costs and time delays. Fortunately, by implementing adaptive design strategies such as the design space (DS) approach, developers can mitigate this risk.
The Design Space Concept
The International Conference on Harmonisation (ICH) and FDA define DS as:
The multidimensional combination and interaction of input variables (e.g., material attributes) and process parameters that have been demonstrated to provide assurance of quality (ICH Q8).4
These regulatory definitions are focused on late-stage development, and the importance of defining a “safe space” within which changes in product and process parameters will not affect clinical performance. The same principles, however, can be applied to early development to maximize the potential to optimize formulations within a single clinical study. Rather than specifying exact values for API and excipient contents, developers can set ranges for these critical-to-performance parameters in the regulatory application. By varying the quantitative levels of these components during clinical conduct, product performance can be influenced and the formulation optimized based on human data. These DS ranges represent acceptable, safe limits based on toxicology studies and PK data collected earlier. Product quality is ensured through inclusion of technical batch data in regulatory documentation. An approval of a complete DS gives developers leeway to adjust formulations as needed within the pre-approved ranges during study conduct. This freedom mitigates risk in MR formulations by giving the Phase I clinical trial process the best possible chance of achieving the desired drug delivery profile – without the need for repeat development cycles.
How Design Space Maximizes Trial Flexibility
The DS concept can be applied to any formulation, drug product, or dosage form. The goal in MR formulations is to address all the adjustable, critical-to-performance parameters that can influence release rate and PK profile. While mapping two variables is common, it is possible to define the DS for as many as are relevant.5 For example, any of these following parameters could be considered as part of the DS:
-API dose/concentration
-Functional excipient content
-Drug:polymers ratio
-Surface area volume ratio
-Coating composition/thickness
Extremes of release rate can be developed in vitro, and the corresponding critical parameter values may be used as the minimum and maximum values to define the DS – the corner points (Figure 1).
Any formulation within this map may be manufactured and dosed without any regulatory amendment or notification. As clinical results develop, trial medications will be adjusted accordingly to optimize the drug-release pattern and increase or decrease the dose as required.
How CRO & CDMO Functional Integration Enables Real-Time Manufacturing & Shrinks Timelines
The flexibility a DS concept affords is only beneficial to the extent that manufacturing can keep pace with the clinical trial dosing modifications. Seamlessly integrating a manufacturing facility with the clinical testing organization running the study can shrink the time between decision points and restart the trial with the next iteration of drug product (Figure 2).
Overall benefits of applying this rapid formulation development and clinical testing approach on demand, as products evolve in the development cycle include the following:
-Timeline shortened by more than 6 months
-Cost of outsourcing reduced by $750,000-$1.5 million
-Formulation selected based on clinical data
-Minimal API waste
-Seamless optimization of formulations for scale-up to commercial manufacturing
Pharmaceutical development teams from more than 50 pharmaceutical and biotechnology companies worldwide have completed more than 100 programs like this while developing optimized MR formulations for small molecule drugs across all major therapeutic areas.
CASE STUDY
Development & Optimization of an MR Formulation Without Relying on Predictive Laboratory or Preclinical Models
A mid-size pharmaceutical company wanted to develop a once-daily format for an existing immediate-release (IR) formulation. An attempt to develop an MR matrix tablet formulation by traditional methods failed to achieve the desired PK profile, and resulted in Cmax related adverse events and sub-optimal therapeutic levels. The company enlisted an innovative CDMO’s help to develop the required formulation using a DS approach with dose and release rate (as determined by HPMC content) as the key variables.
During the development phase, technical batches with 14-day stability data were produced for the four corners of the design space -– the extremes of each variable. These batches were fully characterized using qualified analytical methods in support of the regulatory filing.
Once the application was approved, the clinical PK study in healthy volunteers began, with an initial IR reference period followed by sequential, fasted trials. The initial prototype formulation, within the design space, was determined by PK modelling. Each set of emerging PK data drove selection of the next MR prototype, which was manufactured, quality control tested, and released for dosing without additional approval or stability studies, just days before the next test period. This cycle ended when the PK profile fell within the desired range. Results are shown in Figure 3. One final round was conducted with fed study patients to test the food effect on the PK of the final formulation. This approach identified a tablet prototype with Cmax < 40% and C24 > 200% relative to the IR tablet in 7 months.
Through the integration of formulation design space, real-time GMP manufacturing, and an iterative sequential pharmacokinetic study, an HPMC matrix tablet with desired PK characteristics was developed. Human PK data guided formulation composition, eliminating the need to rely on surrogate, non-predictive laboratory or preclinical data.
OPERATIONAL CHALLENGES: MEETING REGULATORY EXPECTATIONS
To succeed in applying design space concepts to de-risk and expedite clinical trials, scientific and regulatory expertise must go hand in hand. In-depth understanding of the evolving clinical trial regulatory environment will ensure the preparation of high-quality IND applications and institutional review board (IRB) submissions that will satisfy regulatory authorities.
To approve a DS concept, authorities will first need to know how it is mapped: which critical material, process parameters, and/or product quality attributes are the intended variables. The justification package must then provide the following:
-Assurance that systemic exposure of the drug will not exceed that previously demonstrated to be safe and tolerated in humans
-Well-established evidence of safety for all excipients
-Well-established relationship between excipient variables (e.g., molecular weight or levels) and release rate
-Logic of the design space extremes – for example, is the API dose range reasonable based on a sufficient body of supporting information?
-The minimum (therapeutic efficacy)
-The maximum (toxicity/adverse events data)
-Evidence of product quality and stability within the DS to support the duration between manufacture and dosing
To ensure the strongest possible dossier, a collaborative relationship with the IRB is extremely helpful. A CDMO with regulatory personnel who routinely present DS concepts is most likely to achieve a seamless submission and approval process for this type of Phase I clinical trial application – provided that the science and production are impeccable. Successful 14-day make-test cycles demand operational speed and excellence with strong project leadership to integrate clinical and manufacturing activities while managing logistics.
CONCLUSION
Developing MR formulations that fit a target PK profile is far from simple, with many potential delivery technologies to select from and much uncertainty regarding performance in a physiological system. Use of a DS concept saves time and removes uncertainty surrounding achieving the clinical performance of a formulation. With the flexibility to vary the formulation as the clinical trial progresses, a DS concept diminishes risk by increasing the likelihood that the desired PK profile will be achieved relatively quickly, without the need for additional regulatory approval.
An integrated operational set-up allows developers to take maximal advantage of DS concepts through the interplay of manufacturing and clinical testing. Through an iterative make-test cycle, a series of prototypes are rapidly reformulated based on emerging clinical data until the desired performance is achieved. This approach accelerates timelines and greatly diminishes the risk of failing to achieve the desired product.
Advantages of combining a DS approach with real-time, on-demand manufacturing include the following:
-Faster drug development and formulation optimization
-Lower R&D costs
-Lower risk
-Science-based product development
-Seamless transition to Phase II drug product and commercial manufacturing
Enlisting help from a partner with extensive experience in design space, the regulatory expertise to gain approval for them, and the operational capacity to provide real-time manufacturing will ensure successful implementation.
REFERENCES
- Pound P, Ritskes-Hoitinga M. Is it possible to overcome issues of external validity in preclinical animal research? Why most animal models are bound to fail. J. Transl. Med. 2018;16:304. doi.org/10.1186/s12967-018-1678-1.
- Grass GM, Sinko PJ. Physiologically based pharmacokinetic simulation modelling. Adv. Drug Deliv. Rev. 2002;54(3):433-451. doi.org/10.1016/S0169-409X(02)00013-3.
- Musther H, Olivares-Morales A, Hatley OJD, Liu B, Hodjegan AR. Animal versus human oral drug bioavailability: do they correlate. Eur. J. Pharm. Sci. 2014;5:280-291.doi.org/10.1016/j.ejps.2013.08.018.
- USFDA. Conference on Harmonization (ICH) and FDA Guidance for Industry, Q8 (R2) Pharmaceutical Development 2009. https://www.fda.gov/media/71535/download. Accessed May 30, 2019.
- McDermott J, Scholes P. Formulation design space: a proven approach to maximize flexibility and outcomes within early clinical development. Therapeutic Delivery. 2015;6(11):1269-1278. doi.org/10.4155/tde.15.76.
To view this issue and all back issues online, please visit www.drug-dev.com.
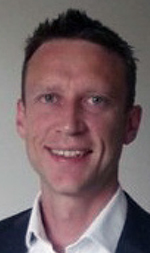
Andy Lewis, PhD, is Vice President, Pharmaceutical Sciences at Quotient Sciences, leading a team of 120 scientists who work on formulation development, clinical manufacturing, and pharmaceutical analysis for clients’ drug product programs. Previously, he was Director of Novel Drug Delivery Technologies at Ipsen (France), where he had global responsibility for product development, utilizing novel formulation technologies or drug delivery devices. He has also assisted in the development and growth of two venture capital funded start-ups, RegenTec and Critical Pharmaceuticals. There he led the development and commercialization of novel technologies in the fields of tissue engineering and drug delivery, taking them from concept into clinical development. Dr. Lewis has a particular interest in overcoming drug delivery challenges, including sustained release and transmucosal delivery of proteins and peptides, and he has filed a number of patents. He is a member of the Academy of Pharmaceutical Scientists of Great Britain; has served for 4 years as the Director-at-Large of the Controlled Release Society (CRS); and is a committee member on the Board of Scientific Advisors.
Total Page Views: 19744