FORMULATION FORUM - A Quick Approach for Evaluation of Drug-Excipient Compatibility: Case of Acetylsalicylic Acid
INTRODUCTION
Drug-excipient compatibility testing is one of the early formulation screening steps that can help predict a drug product’s stability. Conventional methods for compatibility testing however, are time consuming, labor intensive, require screening several excipients and eventually different formulations. For instance, HPLC analysis is often used for compatibility testing taking many weeks if not months to generate data as various blends are held at accelerated conditions.
In contrast, isothermal microcalorimetry may help reduce the timelines for evaluating drug-excipient compatibility in the early stages of formulation development. An indirect and time-efficient approach in predicting and measuring drug instability is possible by measuring interaction energies between formulation components using Thermal Activity Monitoring (TAM). The data generated by TAM may be analyzed in different ways, but for the purpose of simplicity and speed, the Gattefossé application lab uses absolute interaction energies as a singular metric to assess compatibility. This typically involves monitoring the thermal activity of a particular ratio of API: excipient at a constant temperature to that of the API and excipient individually. Any physical or chemical interaction is associated with heat production or absorption which is continuously monitored by TAM. Examples of physicochemical processes that could trigger a calorimetric response include dissolution, adsorption/desorption, evaporation, other phase transitions, crystallization, and chemical reactions. Heat signals are measured for the API and the excipient individually and are used to obtain a theoretical heat signal for the mixture of API and excipient. The theoretical heat signal of the mixture is compared to the measured heat signal. A significant difference between the two is an indication of potential incompatibility.
In this study, we monitor acetylsalicylic acid (ASA) hydrolysis in different formulations using UPLC and TAM with the objective of comparing these methods for agreement, speed, and efficiency in predicting drug stability. In parallel, we assess the impact of the excipient choices on the stability of the ASA.
HYDROLYTIC DEGRADATION & ACETYLSALICYLIC ACID
Hydrolytic degradation is a frequent challenge presented by API and has remained a topic of intense review by researchers.1 Commonly observed with some functional groups, hydrolysis occurs when a chemical bond is split (lysis) in the presence of water. The reaction is highly influenced by atmospheric humidity, pH, and the degree of the reactivity of the functional group. Hydrolysis occurs more rapidly in esters for example than in amides.2
Procaine is an example of an API susceptible to hydrolysis in vivo. Indicated as a local anesthetic, procaine, an amino ester, has short lived effects due to its inherent susceptibility to hydrolysis. Lidocaine, a similar API with local anesthetic properties, on the other hand has longer lasting effects compared to procaine due to its amide structure providing higher hydrolytic stability. Along the same vein, the active methylphenidate (Ritalin), indicated for Attention Deficit Hyperactivity Disorder, contains an ester functional group and is therefore susceptible to hydrolysis. This leads to frequent dosing throughout the day in order to achieve and maintain therapeutic levels of the drug in the blood stream, raising concern for children attempting to comply with dosing recommendations during the day at school.2
Another example of a drug that is subject of hydrolytic breakdown is acetylsalicylic acid. Known for analgesic and antipyretic properties, ASA has been widely used in management of fever and arthritic pain. ASA is also known for inhibitory effect on platelet aggregation, helping reduce infarction risks in patients with cardiovascular disorders.3
Salicylic acid (SA) has a long history of use in the treatment of pain, fever, and inflammation. However, its side effects like severe gastric irritation, nausea, vomiting, bleeding, and ulcers led to the development of ASA.5 In fact, ASA is an esterified pro-drug of the corrosive SA to reduce gastrointestinal irritation and the generation of ulcers.6
Neat ASA is quite stable but becomes susceptible to hydrolysis if exposed to humidity over time (Figure 1) leading to the formation of salicylic and acetic acids.5 The reaction kinetics are relatively slow during storage but rise considerably depending on formulation type and processing conditions. Therefore, it is necessary to avoid hydrolytic changes that would compromise its safety.
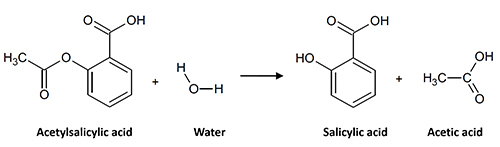
Figure 1. Hydrolytic breakdown of acetylsalicylic acid into salicylic and acetic acids.
The conversion of ASA to SA after oral intake is rapid, which may occur in the GI tract but primarily by enzymatic-mediated hydrolysis in the liver.7 Differences exist between ASA and SA in terms of stability, therapeutic range, and elimination half-life. In pain management, ASA and SA have similar therapeutic effects. In treatment of cardiovascular disease and platelet aggregation however, it is ASA, and not SA, which has the irreversible platelet effect.7
Low dose ASA has been used widely to reduce cardiovascular events and deaths in patients with moderate to high risk of heart disease. However, daily use of ASA leads to increased risk of gastric irritation and serious ulcer complications, hence limiting its use and patient compliance. Lipid based formulations can provide a potential solution by ensuring the release of the API in the duodenum. B. Cryer et. al. demonstrated by a randomized clinical trial that a non-covalent complex of Aspirin- Phosphatidylcholine attenuated low-dose ASA induced ulceration.8
CONTROL OF HYDROLYTIC ACTIVITY
Limiting exposure to humidity during development and manufacturing, addition of buffers to control pH, and protective packaging are common strategies used for mitigating hydrolytic loss of drug activity. Additionally, polymeric coatings may be used as moisture barriers for solid dosage forms. Polymeric coating however requires the use of solvent(s) which need to be subsequently evaporated. Hygroscopic/water soluble polymers are least suited for water sensitive actives, while other polymers will require inorganic solvents which need to be recovered for health and environmental reasons.9 Attractive alternatives to standard polymer coatings are naturally derived lipid-based matrices or coating systems. The latter involve melting of inert, solid lipid excipients before application directly onto the substrate.10 As the lipid solidifies upon cooling, it provides a moisture-resistant barrier for the active. The solvent-free application of solid lipid-based systems can be achieved by several techniques, including holt melt coating, hot melt extrusion, and melt granulation.11
It has been reported that ASA on its own is reasonably stable given its hydrophobic nature resulting in a low level of water sorption.12 ASA crystals tend to generate a hydrophobic field which inhibits the condensation of water from a high humidity environment thus imparting stability to neat API.13 Mitrevej el al. demonstrated that the chemical stability of ASA cannot be improved by combination of the drug with a hydrophilic excipient. Hence, the composition of the formulation plays an important role in maintaining the formulation stability.
Lipid based formulations should be considered when formulating actives like ASA, ibuprofen, and other nonsteroidal anti-inflammatory drugs (NSAIDS) which have the tendency to cause gastric irritation and ulceration. This was demonstrated by a randomized clinical trial conducted by Cryer B. and his team, where 42.2% of subjects treated with a traditional aspirin dosage form (tablets) developed ulcers vs 22.2% of those treated with their lipid-based formulation of ASA.8 For these reasons, this study explores the effect of lipid-based excipients/ formulation type on the stability of ASA while comparing the stability evaluation methods.
METHODS
Lipid formulations: Three formulations containing 32.5% ASA were prepared with four lipid excipients of different phys-chem properties (Table 1).
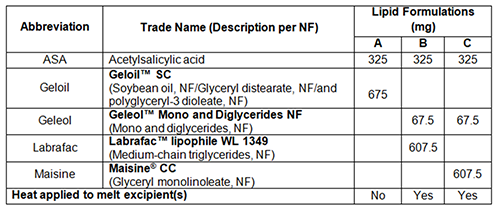
Table 1. Lipid formulations assayed for hydrolytic stability of acetylsalicylic acid.
Geloil™ SC, used in formulation A, is a thixotropic blend of three excipients uniquely developed for softgel formulations at ambient temperature when heat is to be avoided. The product facilitates dispersion of up to 50% solids (API) without loss of flow behavior for capsule filling. Geleol™ Mono and Diglycerides NF on the other hand is a solid excipient in the form of pellets with a melting point of 62°C. This excipient requires melting before mixing with the liquid LabrafacTM lipophile WL 1349 (Formulation B) and/or mixing with the liquid Maisine® CC (Formulation C) prior to dispersing the API in the mixture. Maisine® CC is a widely used oily vehicle, solubilizer and bioavailability enhancer in lipid-based formulations composed of long chain mono, di-, and triglycerides of linoleic acid. Labrafac™ lipophile WL 1349 consists of medium-chain triglycerides of caprylic (C8) and capric (C10) acids. It is used as an oily vehicle and solubilizer in lipid-based formulations.
Two different methods of analysis were employed to trace the ASA changes in various formulations. The first method involved assay of the ASA and its hydrolysis by-product SA using ultra-performance liquid chromatography (UPLC) on aging formulations over a 3-month period. The second method involved the indirect assay of hydrolytic activity by Thermal Activity Monitoring (TAM) over a 10-day period.
UPLC Method: The formulations listed in Table 1 were stored open dish in 250-mL glass beakers at 40°C/75%RH and assayed for SA (hydrolytic degradation product) monthly via a validated reversed-phase UPLC method for three months. As a control, neat API was also stored open dish in 20 ml scintillation vials at 40°C/75%RH and assayed for ASA and SA content monthly for 3 months.
Thermal Analysis Monitoring. TAM studies were conducted under ambient humidity at 40°C for 10 days with individual components and Formulations A, B, and C in sealed glass ampoules. The expected heat flow from a mixture is made up by the contributions in heat flow from the individual components. A difference between the expected heat flow and the heat flow for a real mixture was used to determine the interaction energy and the extent of hydrolysis.
Theoretical signal = Weighted average heat flow signal of components in formulations
Interaction signal = Actual heat signal – Theoretic heat signal
RESULTS & DISCUSSION
The percent assay of ASA over time (in accelerated conditions) was obtained by UPLC method. Even though some trends were evident, the high variability and standard error of the experiments suggested that a more sensitive approach would be to trace % SA, the byproduct of ASA hydrolysis. The results for the latter (Figure 2) proved to be discriminating in terms of ranking the formulations effect on hydrolytic stability.
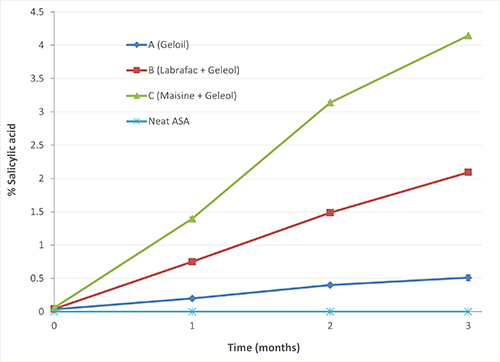
Figure 2. Quantitation of % salicylic acid over three months stability by UPLC.
Based on UPLC analyses, hydrolysis was lowest for formulation A (0.5% SA), given that it did not involve any heat for melting and consisted of a simple dispersion of ASA in Geloil™ SC. Hydrolysis was evident at up to 2% SA for formulation B (LabrafacTM lipophile WL 1349: & Geleol™ Mono and Diglycerides NF) and even higher with formulation C (Maisine® CC & Geleol™ Mono and Diglycerides NF) where ASA was most hydrolyzed (4% SA) (Figure 2). For neat ASA samples stored at 40°C/75%RH conditions, no detectable levels of salicylic acid were generated in three months, confirming that neat API is quite stable as suggested by Mitrevej et al.
In parallel, the same formulations were analyzed immediately after preparation and over a 10-day period under ambient humidity at 40°C using TAM. The interaction energies for formulations A, B, and C after 10 days were 0.23, 1.16, and 5.87 J/g (Figure 3). Evidently, we were able to rank lipid formulations for API excipient compatibility. Similar to the UPLC results, formulation A was most protective of ASA hydrolysis compared to formulations B and C. Clearly, the rank order of hydrolytic breakdown indicated by TAM data is identical to the one obtained using traditional method with UPLC. For formulations which exhibited higher interaction energies, the percent SA at the end of 3 months was also higher.
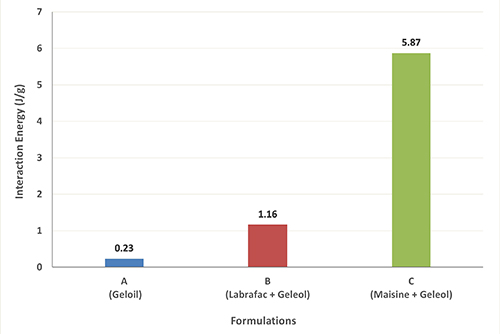
Figure 3. Interaction energy measured by TAM after 10 days.
The differences in the results among the formulations may be explained by the nature of the excipients and the formulation processes used. Formulation A with Geloil™ SC involved simple mixing of ASA with the excipient at ambient temperature. Formulations B and C on the other hand consist of Geleol™ Mono and Diglycerides NF, a solid excipient that needs melting before being mixed with other constituents of the formulation. The idea that the heat involved in formulation preparation could be a potential trigger for API degradation in both B and C is quite plausible. Meanwhile, the higher degradation of ASA in C compared to B may also be due to the nature of the liquid excipients mixed with the solids. However, additional studies to evaluate potential mechanisms are warranted. Overall, the findings are in line with the common understanding that heat should be avoided when working with a sensitive API like ASA.
We note that TAM is an indirect and yet highly sensitive method of predicting interactions in the tested formulations. Significantly less labor intensive than UPLC (validation, standard preparation, sample preparation and analysis) and with quick clues within days into the experimentation, TAM is a powerful discrimination tool in early formulation development.
CONCLUSIONS
The results suggest that the rank order of ASA decomposition C > B> A established by UPLC was further apparent with TAM analysis where the differences between the formulations were more prominent i.e., C>> B>> A. The TAM methodology was faster and less labor intensive.
As a side note, formulation A with Geloil™ SC was more promising compared to the formulations involving heat. Additional studies are needed to demonstrate the direct impact of moisture and heat on hydrolytic stability at a higher temperature and humidity level. Evaluating other API-excipient combinations for compatibility using both TAM and traditional stability testing protocols would further our understanding of the use of TAM in compatibility testing for pharmaceutical dosage forms.
Additional work with Geloil™ SC is warranted with particular attention to inclusion of antioxidant(s), reduced aeration during mixing, and addition of a desiccant to the formulation while measuring water content and acid value at each time point. Future studies to evaluate the in vitro and in vivo performance of both the lipid-based and currently marketed formulations would provide useful insight into the potential of lipid-based excipients in enhancing the overall product performance.
REFERENCES
- LePree, et al. (2013) “Hydrolysis of Drugs”. Ency Pharm Sci and Tech, 4th Ed. Taylor and Francis: NY, 1895-1900.
- Snape et al. (2010) “Understanding the chemical basis of drug stability and degradation”. Pharm J., 285:416.
- Rosenkranz et al. (1986) “Effects of salicylic acid acetylsalicylic alone and in combination on platelet aggregation and prostanoid sysnthesis in man.” Br. J. Clin. Pharmac. 21, 309-317.
- Jannin et al. (2013) “Hot-melt coating with lipid excipients”. Int J of Pharm, 457: 480-487.
- Fersht et al. (1967) “The Hydrolysis of Aspirin. Intramolecular General Base Catalysis” Am Chem Society, 89: 4857-4863.
- Karman Rafik. (2015) From Conventional Prodrugs to Prodrugs Designed by Molecular Orbital Methods. Front in Comp Chem, 2:187-249.
- Dressman J.B. et al. (2012) “Biowaiver Monograph for Immediate-Release Solid Oral Dosage Forms: Acetylsalicylic Acid.” J. Pharm. Sci. 101 (8); 2653-2667.
- Cryer et al. (2010) “Low-dose aspirin-induced ulceration is attenuated by aspirin-phosphotidylcholine: A randomized clinical trial” Am J Gasteroenterol, 106:272-277.
- Yang et al. (2019)” An Update of Moisture Barrier Coating for Drug Delivery”. Pharmaceutics, 11(9):436.
- Jannin et al. (2013) “Hot-melt coating with lipid excipients”. Int J of Pharm, 457: 480-487.
- Becker et al. (2015) “Solvent-Free Melting Techniques for the Preparation of Lipid-Based Solid Oral Formulations.” Pharm Res, 32:1519-1545.
- El-Banna et al. (1978) “Aspirin stability in solid dispersion binary system” J. Pharm. Sci.,1631: 1633.
- Mitrevej et al. (1983) “Influence of hydrophilic excipients on the interaction of aspirin and water”. Int. J. Pharm., 243:250.
Dr. Masumi Dave is Pharmaceutical Application Lab Manager, at Gattefossé USA where she manages a team of analytical and formulation scientists, overseeing R&D projects. The focus of the lab is to characterize, design, and develop novel approaches to the use of lipid-based excipients with the overall goal of providing technical support to pharmaceutical companies. She began her career at Gattefossé USA in 2017 as Application Lab Scientist, following completion of her PhD and Master’s in Pharmaceutics at Long Island University, which was preceded by a Bachelor’s degree in pharmacy at Rajiv Gandhi University in 2007.
Rollie Fuller is Technical Service Coordinator at Gattefossé USA, where he is responsible for accurate customer follow-up with provision of extensive technical and regulatory documents and information relating to Gattefossé’s pharmaceutical excipient product offerings. With an expertise in a wide array of application areas, the Technical Service Department provides assistance for both internal laboratory projects as well as external technical support. He began at Gattefossé USA in 2019. He completed his Bachelor’s degree in Chemical and Biomolecular Engineering from New York University in 2017.
Dr. Jason LePree earned his BS in Pharmacy from Rutgers University and his MS and PhD in Pharmacy (Pharmaceutics) from the University of Wisconsin-Madison. He has over 20 years of experience in both Academia and Industry, where he held leadership positions in preformulation, formulation, analytical R&D, and quality control. In parallel, he has served as Adjunct Professor at Long Island University since 1998, instructing on Physical Chemistry, Pharmacokinetics, and Pharmaceutical Analysis.
Total Page Views: 7153