CELL SEPARATION TECHNOLOGY - Breaking Down Barriers to Unlock a New Era of Cell Therapy
INTRODUCTION
Cell therapy has, for many years, held the promise of revolutionizing the treatment of cancer and other diseases by harnessing the power of the patient’s own immune system and providing an effective last-resort cure where other treatments have failed. However, until recently, high production costs and inefficient processes have hampered the field. Now, recent advancements are helping to remove these barriers to R&D and are ultimately making treatments, such as CAR-T, CAR NK, and stem cell therapies, more accessible than ever to patients. CAR-T cell immunotherapy, in particular, has shown promising results in treating blood cancers – including multiple myeloma and certain forms of leukemia and lymphoma – whereby T cells are first extracted from a patient’s blood, and then genetically modified in a laboratory to express a chimeric antigen receptor (CAR).1 Once infused back into the patient, these modified T cells target the cancer cells expressing the specific protein recognized by the CAR. This approach to treatment helps to minimize the damage to normal, non-cancerous cells, causing fewer side effects and leading to more effective treatment.
POINT-OF-CARE AUTOLOGOUS CELL THERAPY MANUFACTURING
Current CAR-T cell production for these therapies is largely centralized, with healthcare centers sending patient samples to specialized manufacturers, but the situation is far from ideal. Unfortunately, transporting cells and final products between healthcare providers and producers requires cold chain storage, incurring high logistics costs. The wait time between initial cell harvest and the receipt of the therapy back at the hospital can also be in the order of several months, delaying the onset of critical treatment. Decentralized manufacture of autologous CAR-T cells closer to the point of patient need – for instance in a hospital laboratory – would address some of these challenges, starting with avoiding long-distance transportation and the associated expenditure. The turnaround time from cell harvesting to the administration of therapy would also be much shorter, potentially as little as 3 days, enabling earlier and more effective treatment. There is therefore a trend in both North America and Europe for larger medical institutions to manufacture their own cell therapy products on site according to good manufacturing practices (GMP) instead of outsourcing production. Regulatory agencies are also recognizing the difference between cell therapy and conventional drug products, supporting the development of novel advanced therapies by offering healthcare institutions that satisfy rigorous GMP and quality control requirements an exemption and enabling easier access to cell therapy for patients.2
STREAMLINING CAR-T CELL MANUFACTURE
The actual process of traditional CAR-T cell manufacturing itself can take up to 12 days from initial harvest, including T cell isolation and activation, gene transduction and ex vivo expansion. This expansion process is extremely resource intensive and costly, and also exhausts CAR-T cells, causing them to lose their potency and efficacy as a cell therapy. Recent studies have shown that the ex vivo expansion process is, in fact, not strictly necessary in many cases, as CAR-T cells are capable of proliferating by themselves in the body.3,4 Direct infusion of CAR-T cells into the bloodstream would therefore preserve their potency and allow therapies to be delivered to patients sooner. Bypassing the lengthy expansion phase would also significantly reduce overall manufacturing costs, encouraging wider production and adoption of cell therapies. For these reasons, many major biopharma players and large medical institutions are now implementing rapid manufacturing processes that take as little as 3 days and are more cost effective than previous production methods.5,6
STRIKING THE RIGHT BALANCE BETWEEN CARE & COST
Outside of manufacturing, the overall cost of treatment to a healthcare provider is largely wrapped up in the resources required for long-term patient care. The US FDA requires that patients are pre-treated and well monitored before a full dose of cell therapy is administered in order to avoid any adverse effects such as cytokine release syndrome. This condition is severe and usually leads to prolonged stays in the intensive care unit (ICU), significantly increasing overhead and resource costs for the hospital. In some trials, lower doses of more potent CAR-T cells have been shown to elicit a far milder form of cytokine release syndrome, with only a very small percentage of patients suffering from the condition in comparison to those treated with higher doses.7 Using smaller doses of more potent CAR-T cells – produced through rapid manufacturing processes and administered directly – could help to prevent ICU admissions and shorten hospital stays. This in turn would contribute to reducing the financial burden of treatment to healthcare providers.8
OVERCOMING HURDLES IN CELL SEPARATION
There are understandably still some challenges that must be overcome to ease the transfer of cell therapy production from specialized to healthcare facilities. T cell separation is a critical upstream process within CAR-T cell manufacturing and has a significant impact on the quality of the cells produced, subsequently affecting the ultimate success of the therapy. Separating T cells from blood with adequate levels of purity, viability, and concentration can be difficult, with the technical limitations of conventional cell separation techniques – such as density gradient centrifugation, column-based magnetic cell separation, and fluorescent activated cell sorting (FACS) – significantly hindering research capabilities. When employing these methods, multiple platforms are typically needed for each sample preparation, extraction, engineering and culture stage, all with their own single-use components and costly reagents. These set-ups are therefore not only expensive for researchers and manufacturers, but also occupy a lot of space and require constant manual input. In addition, current cell separation and enrichment protocols often result in suboptimal purity, yield, and cell viability, potentially limiting patient access to state-of-the-art drugs. These shortfalls are driving researchers and manufacturers in the biopharma and healthcare spaces to improve the efficiency of cell separation processes for enhanced purity and yield.
Powerful automated solutions now exist for rapid, column-free immunomagnetic cell separation (Figure 1). These platforms use matrix-free magnetic technology to reduce dead volume and cell trapping, isolating cells with high purity and recovery rates (Figure 2). This method also does not use centrifugation, treating cells gently to preserve their viability and physiology for downstream functional studies. Systems based on this methodology are compatible with a variety of input sample types, including peripheral blood, bone marrow, and apheresis products, and do not require cell washing before or after labelling, greatly simplifying the cell separation workflow. Features such as automated three-pass cell enrichment also confer consistent separation between runs, making it possible to process billions of cells in a single closed system operation. This capacity increases throughput and enables users to avoid slow and laborious manual methods, allowing them to just walk away and get on with other tasks. The addition of options, including sterilizable, reusable fluidics along with economical, GMP-compliant consumables, is also helping to reduce per sample running costs and contribute to cost-effective R&D and scale-up.
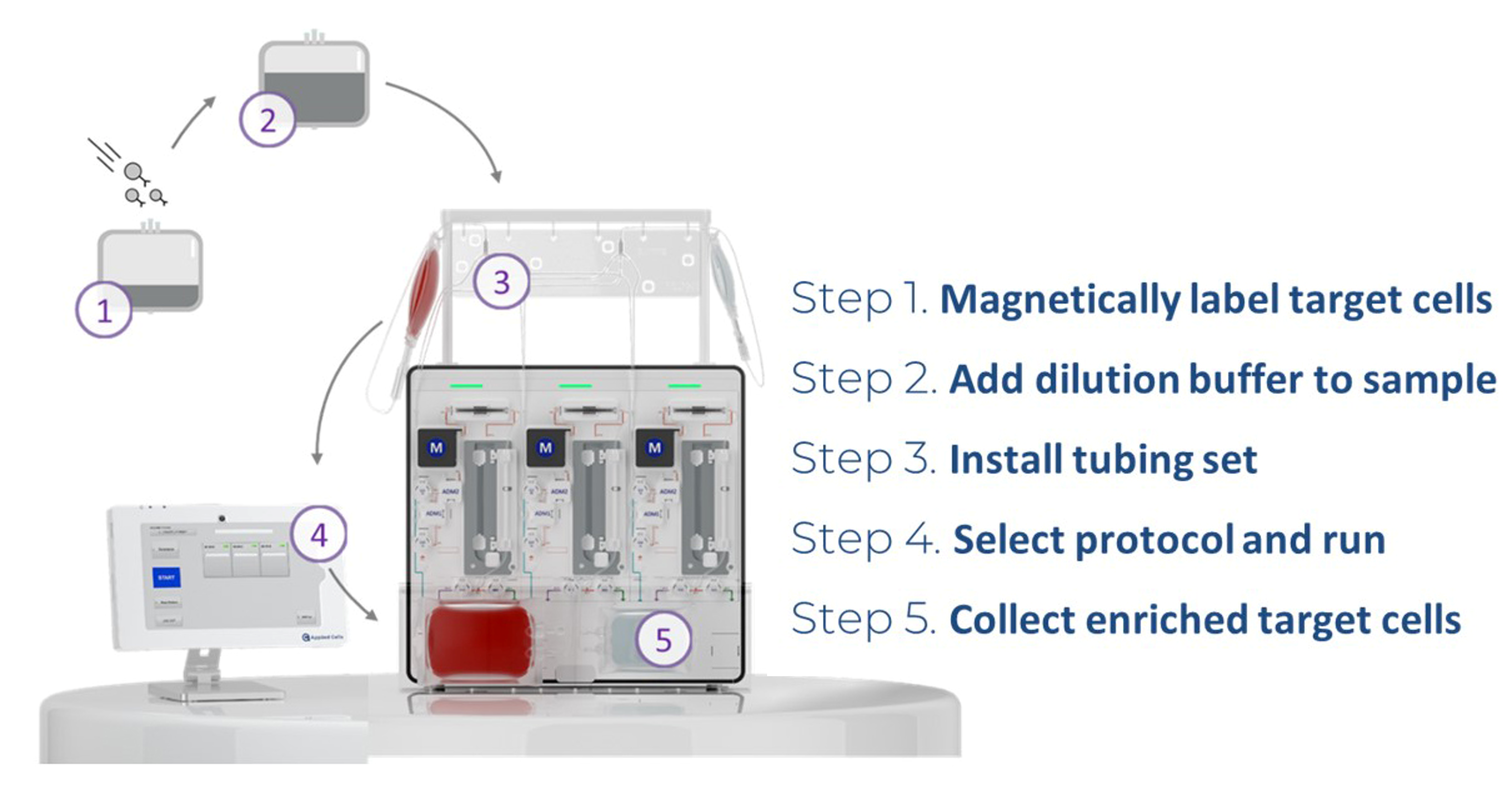
Figure 1. The MARS® Bar platform from Applied Cells uses column-free sterile fluidic technology and offers a convenient and affordable method for isolating T cells in a closed system.
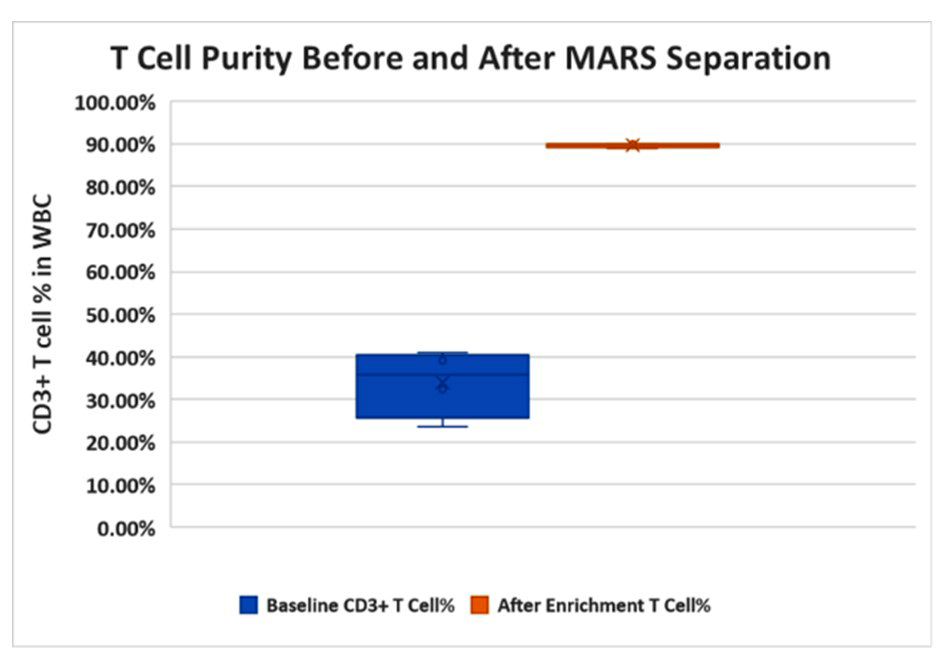
Figure 2: T cells are enriched up to 90% percent purity on average after separation on the MARS Bar platform.
The development of compact platforms based on this technology that can easily be adapted for use in both research and manufacture, where the same protocols can be applied across all development stages, is effectively streamlining and standardizing the transition from R&D to commercial and decentralized production. This avoids the need to purchase extra platforms for scale-up and allows manufacturers in biopharma and healthcare to circumvent additional process development after initial set-up and optimization.
AN ENCOURAGING OUTLOOK FOR PERSONALIZED MEDICINE
Autologous CAR-T cell therapies have already been successful in treating hematological cancers, accelerating research into their applications for a broad range of other disease areas. In fact, multiple clinical trials investigating the efficacy of these immunotherapies for treating autoimmune diseases, such as systemic lupus erythematosus, and hereditary disorders including cystic fibrosis, are currently underway, and are already showing promising results.9 Revolutionary cell separation technologies are crucial for enabling these rapid advancements, and will continue to play a growing role in establishing the decentralized, rapid, and cost-effective large-scale manufacture of potentially life-saving cell-based drugs well into the future.
*For research use only. Not for use in therapeutic or diagnostic procedures.
REFERENCES
- National Cancer Institute. CAR T Cells: Engineering Patients’ Immune Cells to Treat Their Cancers. National Institute of Health .
- Giorgioni L, Ambrosone A, Cometa MF, Salvati AL, Magrelli A. CAR-T State of the Art and Future Challenges, A Regulatory Perspective. Int J Mol Sci. 2023;24(14):11803. doi:10.3390/ijms241411803
- Ghassemi S, Nunez-Cruz S, O’Connor RS, et al. Reducing Ex Vivo Culture Improves the Antileukemic Activity of Chimeric Antigen Receptor (CAR) T Cells. Cancer Immunol Res. 2018;6(9):1100-1109. doi:10.1158/2326-6066.CIR-17-0405
- Ghassemi S, Durgin JS, Nunez-Cruz S, et al. Rapid manufacturing of non-activated potent CAR T cells. Nat Biomed Eng. 2022;6(2):118-128. doi:10.1038/s41551-021-00842-6
- Yang J, He J, Zhang X, et al. Next-day manufacture of a novel anti-CD19 CAR-T therapy for B-cell acute lymphoblastic leukemia: first-in-human clinical study. Blood Cancer J. 2022;12(7):104. doi:10.1038/s41408-022-00694-6
- Dickinson MJ, Barba P, Jäger U, et al. A Novel Autologous CAR-T Therapy, YTB323, with Preserved T-cell Stemness Shows Enhanced CAR T-cell Efficacy in Preclinical and Early Clinical Development. Cancer Discov. 2023;13(9):1982-1997. doi:10.1158/2159-8290.CD-22-1276
- Ayala Ceja M, Khericha M, Harris CM, Puig-Saus C, Chen YY. CAR-T cell manufacturing: Major process parameters and next-generation strategies. Journal of Experimental Medicine. 2024;221(2). doi:10.1084/jem.20230903
- Ayala Ceja M, Khericha M, Harris CM, Puig-Saus C, Chen YY. CAR-T cell manufacturing: Major process parameters and next-generation strategies. Journal of Experimental Medicine. 2024;221(2). doi:10.1084/jem.20230903
- Blache U, Tretbar S, Koehl U, Mougiakakos D, Fricke S. CAR T cells for treating autoimmune diseases. RMD Open. 2023;9(4):e002907. doi:10.1136/rmdopen-2022-002907.
Dr. Liping Yu is VP of Applications at Applied Cells, overseeing application development, providing customers with complete solutions by integrating reagent, hardware, and software on the MARS platform. She earned her PhD from Carnegie Mellon University in 2006 and then undertook postdoc training and career development at Beckton Dickinson. She is an experienced team leader with track record of strategic planning and customer engagement, having overcome significant business and technical challenges to deliver a successful product from concept to production, and led a versatile team spanning across the company’s organizational boundaries through technology development. She has additionally established a large number of close and collaborative relationships with academic and industrial partners.
Total Page Views: 461